Introduction to Physical Geology |
|
Chapter 2 - Physical Properties of Earth Materials |
2.1
This chapter is divided into two sections:
1) Important concepts in chemistry and physics (sections 2.2 to 2.9), and
2)
An introduction earth materials: rocks and minerals (sections 2-10 to 2-40).
The first section provides an introduction to concepts that are important to all physical sciences. Basic concepts of chemistry and physics are fundamentals as they relate to the study of materials and processes occurring on Earth and other objects in space. Important chemistry concepts include general knowledge about atoms, elements, chemical compounds, chemical formulas, the nature of chemical bonds. Physics topics include information about mass & density, electricity, magnetism, energy, and gravity. All these topics are revisited in applied to geology discussions in following chapters.
The second part of the chapter focuses on rocks and minerals, the natural solid materials that make of the rocky planets like Earth. All natural materials are made up of elements that form compounds that have unique properties related to how they form in the natural environment. Everything around us is made of chemical compounds that have testable and identifying characteristics that allow them to be classified. This applies to rocks, minerals, and derivative materials (such as sediments and soil). Minerals are the crystalline chemical compounds that combine together to for rocks.
|
Click on images for a larger view. |
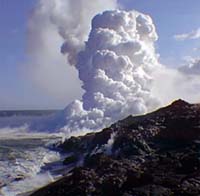
Fig. 2-1. All earth materials form from the interactions of matter and energy. |
2.2
Important Concepts In Chemistry and Physics
The following sections provide basic background information that are essential to understanding the physical and chemical properties of matter, particularly related to natural earth materials (rocks, seawater, air, organic matter, etc.).
The chemical characteristics of earth materials reveal information about the environments how and where they are formed, Their characteristics also determine their potential fate when exposed to chemical changes over time. For instance, rocks formed deep underground may not be stable in the surface environment where they are exposed to water, air, temperature changes, or other changing physical and chemical conditions.
Basic chemistry concepts needed to be understood for this geology course include:
|
* All matter is made up of atoms, and atoms are made up of atomic particles (electrons, protons, and neutrons; Figure 2-2). An atom is the smallest unit of a chemical element. Atoms have a nucleus composed of neutrons & protons and has a positive charge. Negatively charged electrons orbit around the nucleus in shell-like layers.
|
* A chemical element is a pure chemical substance consisting of one type of atom distinguished by its atomic number, which is the number of protons in its nucleus. Elements have equal balance in numbers of positively charged protons and negatively charged electrons. Common examples of elements are iron, copper, silver, gold, hydrogen, carbon, nitrogen, and oxygen.
|
* An element is a substance that cannot be broken down into simpler substances by chemical means.
|
* An element is composed of atoms that have the same atomic number, that is, each atom has the same number of protons in its nucleus as all other atoms of that element. Each element is assigned a 1-2 letter symbol to represent the element for general use, such as in the writing of chemical formulas (such as H2O used for water).
|
* The periodic table is a list of known chemical elements arranged in order by atomic number from smallest to largest and by group chemical properties (Figure 2-3). The periodic table is a list of 108 known elements. Of these, 92 are naturally occurring elements (prior to development of artificial nuclear research and development). The lightest element, hydrogen, has one proton, whereas the heaviest naturally occurring element, uranium, has 92 protons. In general elements on the left side of the periodic table (Figure 2-3) are elements classed metals (highlighted in gray, green, yellow, and pink), and elements on the right (shown in blue) are nonmetals. On the far right in orange are a group of elements know as noble gases.
|
* Atoms bond together to form molecules. A molecule is a group of atoms bonded together, representing the smallest fundamental unit of a chemical compound that can take part in a chemical reaction. For instance, a molecule of water—chemical formula, H2O —is made up of two hydrogen atoms and one oxygen atom (Figure 2-4).
|
* A chemical compound is a pure chemical substance consisting of two or more different chemical elements that can be separated into simpler substances by chemical reactions. Chemical compounds have a unique and defined chemical structure; they consist of a fixed ratio of atoms that are held together in a defined spatial arrangement by chemical bonds. All minerals are chemical compounds, but by comparison relatively few compounds are naturally occurring minerals!
|
*
Types of molecular bonds (chemical forces that hold molecules together) include metallic (for metals), ionic (compounds that dissolve easily), covalent (most others). Chemical bonds are discussed below.
|
* A mixture is a combination of two or more pure substances in which each pure substance retains its individual chemical properties. Examples of mixtures include rocks, magma (molten rock) air, and seawater.
|
* Chemical formulas are used to describe compounds such as H2O (for water), NaCl (for salt), CO2 (for carbon dioxide). Chemical formulas may be simple text designations showing the ratio of elements, or may be represented by graphic means showing relationships (orientation and bonding) between elements within molecules, as illustrated with caffeine in Figure 2-5. |
|
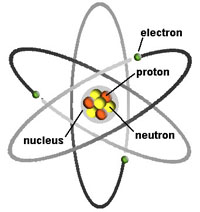
Fig. 2-2. A conceptual view of an atom. Atoms are composed of protons, neutrons, and electrons.
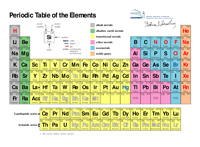
Fig. 2-3. The periodic table of the elements is an arrangement of the elements based on atomic number (number of protons in an atom).
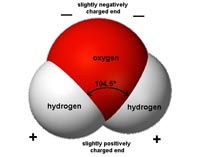
Fig. 2-4. Graphic illustration of a water molecule composed of two hydrogen atoms bonded to an oxygen atom.
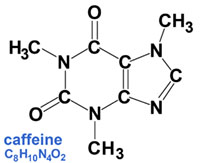
Fig. 2-5. Chemical formulas for the caffeine molecule (text and illustration versions). |
2.3
Chemical Bonds
Molecular compounds are held together on an atomic level by chemical bonds. Chemical bonds are persistent forces of attraction between atoms or molecule caused by electrostatic forces (positive or negative charges) or the sharing of electrons between bonded atoms. Three types of chemical bonds include ionic bonds, metallic bonds, and covalent bonds. The types of chemical bond influence the physical properties of the molecular compounds they form.
Ionic Bonds
Molecular compounds held together by ionic bonds are salts. An ionic bond is a chemical bond between two oppositely charged ions. Typically, metals lose valence electrons (loose electrons in their outer shell of orbiting electrons) to become positively charged cations, whereas the nonmetal accepts electrons to become negatively charged anions. For example, common salt (NaCl) has ionic bonds between sodium (Na+) has a positive charge and chlorine (Cl-) has a negative charge. Salts readily dissolve in water as their charged ions are attracted to parts of water molecules that can also have positive and negative charges. As water evaporates, the ions dissolved in water will precipitate again as salts (Figures 2-6 and 2-7). Natural salts like halite (NaCl) and gypsum (CaSO4) are generally soft minerals and can dissolve in water.
Metallic Bonds
Metals are held together by metallic bonds. Compounds with metallic bonds transmit electricity. With metallic bonds, the electrons disassociate from orbiting a single atom and become more of a cloud electrons that surround the positively charged nuclei of interacting metallic ions. Metalloids are intermediate between those of metals and solid nonmetals. Although most elements are metals (all those on the left and center parts of the Periodic Table), only a few elements occur naturally in metallic form including gold, platinum, copper, iron, and mercury (in liquid form). Some minerals are metalloid compounds including pyrite (FeS2), magnetite (Fe3O4), and galena (PbS) (Figure 2-8).
Covalent Bonds
Molecular compounds held together by covalent bonds are non-metallic compounds. Covalent bonds occur when two or more atoms share orbiting electrons, creating more stability in the valence shell of electrons between the bonding elements. These materials can form crystal complexes and do not transmit electricity and tend to be harder, more durable compounds. For instance, most gem minerals are non-metallic compounds with covalent bonds. The mineral quartz (SiO2) is a non-metallic crystalline compound (see Figure 2-9).
Van der Waals force
Van der Waals forces (bonds) are weak, nonspecific forces between molecules and include attractions and repulsions between atoms, molecules, and surfaces.
Van der Waals forces are responsible for friction and what makes water sticky.
|
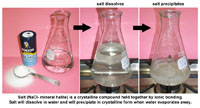
Fig. 2-6. Salt crystals are held together by ionic bonds. Salt compounds dissolve in and precipitate from water.
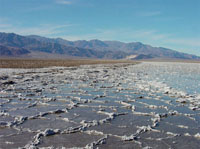
Fig. 2-7. This view shows salt crystals precipitating on a dry lakebed in Death Valley, California.
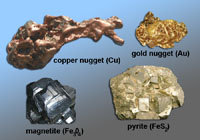
Fig. 2-8. Metallic bonds occur in metallic minerals (like native copper and gold) and metalloid minerals (like magnetite and pyrite).
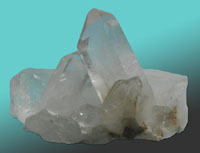
Fig. 2-9. Most minerals are non-metallic crystalline compounds held together by covalent bonds (and will not transmit electricity). [This mineral sample is quartz.]
|
2.4
Isotopes (and Radioactivity)
Many elements have one or more isotopes. Isotopes are of the same element that contain equal numbers of protons but different numbers of neutrons in their nuclei, and hence differ in relative atomic mass but not in chemical properties. Some isotopes are not stable and ultimately break down or change into other elements. We call such isotopes radioactive. Many elements have both stable and radioactive isotopes. For example, the element carbon has 3 isotopes: 12C and 13C are stable, whereas 14C is unstable and will undergo radioactive decay. All there isotopes have 6 protons, but have 6, 7, and 8 neutrons, respectively.
In the natural environment there are 80 different elements that have one or more isotopes. Of these, at least 254 stable isotopes that have never been observed to decay. Another 50 isotopes are radioactive (these isotopes are called radionuclides). In most naturally occurring materials the amount of radioactive isotopes is relatively insignificant in measurable concentrations (Figure 1-15). However, with the invention of nuclear weapons, and the numerous nuclear bomb test through the 1950s to the present, and accidents involving poorly designed nuclear power plants, there are now many more radioactive isotopes loose in the environment. The mixing of these radionuclides in the air, water, and sediments dilute their concentrations, but also disperse them to all regions of the world.
For example, the 2011 Fukushima Daiichi nuclear disaster
associated with the massive earthquake and tsunami in Japan released large amounts of radiation into the marine environment around Japan.
The Chernobyl disaster of 1986 in the Ukraine also released large amounts of radioactive material into the region and atmosphere. |
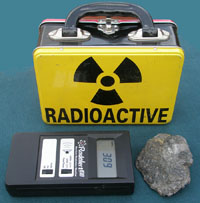
Fig. 2-10. Radioactive elements that occur in rocks and minerals include isotopes of potassium, thorium, radium, and uranium. and may display measurable radioactivity. A geiger counter us used to measure materials for radioactivity.
|
2.5
Mass and Density
Mass is the property of matter that measures its resistance to acceleration. Gravity is an accelerating force, but machines like cars or rockets can create artificial acceleration. Roughly, the mass of an object is a measure of the number of atoms within it.
Mass is often confused with weight. Weight is a measure of an amount of mass under the influence of gravity. For instance, a 150 pound person on Earth would only weigh 25 pounds on the moon because the Moon only has 1/6 the gravity of Earth.
Density is the ratio between mass and volume. It is a measure of how much matter an object has in a unit volume (such as cubic meter or cubic centimeter).
• Density = mass/volume
• Usually defined in grams per cubic centimeter (gm/cm3),
• or as measured for liquids as grams per milliliter (gm/ml). 1 ml = 1 cm3.
Density Stratification
• The Earth's internal structure, oceans, and atmosphere have layers based upon density differences; they are density stratified. For instance, ice is less dense than freshwater, and freshwater is less dense than seawater. As a result, ice will float on freshwater, and without mixing, freshwater will float on seawater.
Pure water at room temperature (about 70° Fahrenheit) is 1.0 gm/cm3 or 1.0 gm/ml.
Seawater has an average density of 1.027 gm/cm3, but this varies with temperature and salinity over a range of about 1.020 to 1.029 gm/cm3.
What is the change in density when we add 1% salt to freshwater? Calculation:
(0.99)(1.0 gm/cm3) + (0.01)(3.0 gm/cm3) = 1.02 gm/cm3 |
Examples of the density of earth materials
:
• Air: ~0.1 gm/cm3
• Freshwater: 1.0 gm/cm3
• Ice: 0.917
gm/cm3
• Saltwater: ~1.001-1.03 gm/cm3 (average 1.027 gm/cm3)
• Surface rocks: ~2.7-3.3 gm/cm3 (average ~3 gm/cm3)
• Matter at the center of Earth: ~16 gm/cm3
|
Density of common materials:
• Wood: ~0.6-0.9 gm/cm3
• Glass: ~2.4 gm/cm3
• Aluminum:
2.79 gm/cm3
• Iron: 16 gm/cm3
• Copper: 8.96 gm/cm3
• lead: 11.36 gm/cm3
• Gold: 19.32 gm/cm3
|
The average density of a human is about 0.986 gm/cm3 -- when holding one's breath while in water the average human density decreases to about 0.945 gm/cm3. Because seawater is slightly denser than freshwater, humans float slightly higher in seawater over freshwater.
|
What is the difference between mass and weight?
Mass is a measure of the actual amount of material contained in a body and is measured in grams, pounds, etc.
Weight is the force exerted by the gravity on that object.
For instance, an astronaut weighing 180 pounds on Earth weighs only 30 pounds on the Moon because the Moon has only about 1/6 the gravity of Earth. |
2.6
Electricity and Magnetism
Electricity and magnetism are separate phenomena related to a singular underlying force called electromagnetism. Electricity is related to atomic charges carried by electrons (negative) and protons (positive). The charges are equal but opposite, but protons have mass nearly 1,830 greater than electrons. (Neutrons have the same mass as protons, but have no electrical charge). As a result, electrons are freer to move than protons (hindered by their greater mass).
Electromagnetism is a force generated between electrically charged particles on a subatomic level. Within atoms, positively charged protons are trapped in the nucleus, whereas negatively charged electrons are in motion outside the nucleus. Electrons move in static shell-like orbits around the positively charged nucleus. Electrons are attracted to the positive forces of atomic nuclei (protons) but are repelled by other electrons (basically, opposites attract, similarly charged particles repel each other).
Under the right conditions, with certain types of matter (such as iron in magnets, copper wires, and all substances that are metallic in nature) allow electrons to flow between atoms—this flow creates an electrical current (electricity). This flow of electrons generates a magnetic field, with the intensity of the magnetism generated is proportional to the volume or intensity of electrical flow. A magnetic field extends onto space beyond the matter generating the electrical current (Figure 2-11). As a result, magnets have positively and negatively ends—oppositely charged ends attract each other, where as similarly charged ends repel each other.
The Earth and other planets have special characteristics that allow them to generate great magnetic fields that extend into outer space (Figure 2-12). A compass is an instrument containing a pointer composed of a magnet that aligns itself with Earth’s magnetic field, pointing in the direction toward Earth’s magnetic north pole. Earth's magnetic field is preserved in iron-bearing rocks when they form. Earth's magnetic field is also responsible for the aurora borealis (Northern Lights) and aurora australis (Southern Lights)(discussed in Chapter 1, section 1-27). |
Fig. 2-11. Magnetic field around a magnet revealed by magnetic sand on a layer of paper.
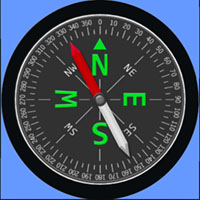
Fig. 2-12. A compass arrow always points toward Earth’s magnetic north pole. |
2.7
The Electromagnetic Spectrum
Electromagnetic energy is the release of energy is in the form of quantum particles called photons (for example, a light beam is a stream of photons).
[Quantum is defined as a discrete quantity of energy that is proportional in magnitude related to the vibrational frequency of electromagnetic radiation.]
● Photons are quantum features that have no mass but behave both like a vibrational wave and a particle, simultaneously. Photons transmit both energy and momentum.
● Photons travel at the speed of light, which in the vacuum of space is about 2.998 x 108 meters per second, or 186,000 miles per second.
● A discrete amount of energy is associated with a photon and is proportional to the vibrational frequency and wavelength of the electromagnetic wave—the faster the vibrational frequency, the shorter wavelength and the higher the energy transmitted.
● Photons can be destroyed or created when they are absorbed or emitted from atoms or atomic particles with mass. On a subatomic level, electromagnetic energy is either released or absorbed as electron orbits shift closer or further from the atomic nucleus.
● Photons move in a linear motion, such as illustrated by a ray of light radiating away from a light source.
Electromagnetic radiation are forms of energy that can be emitted or reflected from objects through electrical or magnetic waves traveling through space. The electromagnetic spectrum describes the different forms of electromagnetic radiation ranging from low frequencies with long wavelengths (such as radio waves) to very short wavelength, high energy gamma rays (Figure 2-13). The shorter the wavelength, the greater the amount of energy is transferred by an electromagnetic wave. All natural materials either transmit, reflect, or absorb electromagnetic energy in different ways.
|
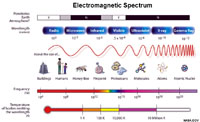
Fig 2-13. The electromagnetic spectrum is the range of wavelengths or frequencies over which electromagnetic radiation extends. Visible light is only a small portion of the electromagnetic spectrum. Infrared radiation (IR) are the invisible heat rays we can feel being released by hot objects, such as the warmth of sunlight. Ultraviolet light, X-rays and cosmic rays have increasing shorter wavelengths and higher energy than visible light. Infrared radiation, microwaves, and radiowaves have increasingly longer wavelengths and are lower energy than visible light. |
2.8
Energy
Energy is the capacity for doing work. Energy may exist in various forms: chemical, electrical, nuclear, potential, kinetic, and other forms. Energy is integral to all processes taking place in our physical environment ranging from incoming sunlight to the movement of wind and water, weather, earthquakes, volcanic eruptions.
All physical and chemical reactions involve either the loss or gain of some form of energy.
The Sun's electromagnetic energy radiates from nuclear fusion processes in the Sun's core through to it's surface before radiating into space as solar energy. The immense pressure in the Sun's core fuses hydrogen atoms into helium atoms—a process that gives off vast amounts of energy and causes the Sun and other stars to glow. Solar energy is electromagnetic radiation (mostly visible light). Some of the light is absorbed by the atmosphere, oceans, and land is converted to heat or other forms of energy. Much of what the Earth receives is reflected back into space. An equivalent amount of solar energy is radiated daily back into space; only a trace of solar energy is trapped by plants and to support life in the process over time. The exchange of solar energy is the kinetic driving force behind all motion in Earth's atmosphere and oceans. Solar energy trapped in Earth's tropical regions heats the oceans and air which drives the movement of the global atmospheric and oceanic circulation systems moving heat toward the polar regions.
Geothermal energy is the driving force for motion within the planet (including deep mantle currents that drive plate tectonics motion, and is ultimately the source of energy released in earthquakes, volcanoes eruptions, and hot springs and geysers, Figure 2-14). Geothermal energy released from the Earth's surface comes from heat trapped deep inside the Earth left over from the formation of the planet (about 5 billion years ago). Geothermal heat is also generated by the decay of radioactive elements, most of it from long ago. Both solar electromagnetic energy and geothermal energy are utilized to support life and ecosystems within the Earth' s marine and terrestrial environments.
|
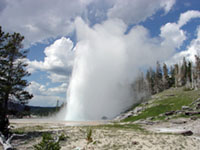
Fig. 2-14. Geothermal energy is released from the planet in a variety of ways, including heat associate with volcanoes, or the release of hot water associated with hot springs and geysers. The amount of heat migrating to the surface varies from place to place and may be less or greater than heating from incoming solar energy. This view is Grand Geyser erupting in Yellowstone National Park, Wyoming.
|
2.9
Gravity
Gravity is a mysterious force that attracts a physical body toward the center of the Earth, or toward any other physical body toward any other body having mass (such as planets orbiting around the Sun, or moons around a planet). Gravity is a accelerating force, meaning that it's effects are similar to a measurable change in velocity. On the earth surface, the average rate of gravitational acceleration is an increase of about 9.8 meters per second per second (m/sec2).
Understanding the very mysterious force of gravity is fundamental to characterizing the mechanics of the orbits of planets and moons within the Solar System (and objects moving throughout the universe). Using research by earlier astronomers, between 1609 and 1619, Johannes Kepler presented scientific laws that describe to character of the elliptical motion of planets around the sun. Isaac Newton used Kepler's laws to mathematically resolve the nature of gravity which he presented in 1687 as his Law of Universal Gravitation which states "a particle attracts every other particle in the universe using a force that is directly proportional to the product of their masses, and inversely proportional to the square of the distance between them" (Figure 2-15). Gravity is a weak but measurable force, but becomes observable when dealing with objects on the scale of moons, planets, and satellites launched into space.
The force of gravity actually varies from place to place. This is due to the variations in the density of rocks preserved within the Earth's crust. For instance, rocks under the ocean basins are more dense that rocks found beneath most continental regions. Small variations in gravity are measured using a device called a gravitimeter. In addition, detailed measurement in the flight paths of satellites orbiting the planet have been used to measure variations in the Earth's gravitational field. |
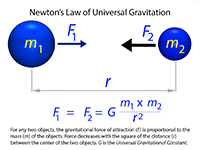
Fig. 2-15. Newton's Law of Universal Gravitation. All matter is subject to gravitational forces associated with other matter. Even though gravity is a very weak force, with increasing mass of an object, the force of gravity it produces also increases. Gravity is the measurable force that holds planets and moons in orbit in the Solar System. |
2.10
What are the most abundant elements in Earth's physical environment?
The most abundant elements in our physical environment are: H, C, N, O, Na, Mg, Al, Si, P, S, Cl, K, Ca, Fe
See their locations on the Periodic Table, Figure 2-3.) In chronological order by atomic number, these symbols are H hydrogen, C carbon, N nitrogen, O oxygen, Na sodium, Mg magnesium, Al aluminum, Si silicon, P phosphorus, S sulfur, Cl chlorine, K potassium, Ca calcium, and Fe iron. Be prepared to name these elemental symbols! (Figure 2-16). These elemental symbols are used in discussions below and throughout the following chapters.
These elements are:
* ingredients of common minerals, rocks, sediments, and soil (solids)
* components of seawater and air (liquids & gases)
* essential nutrients for life (organic compounds). |
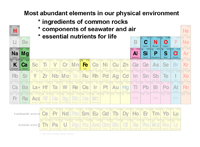
Fig. 2-16. Most abundant elements in the physical environment. |
2-11
What is the elemental composition of the Earth's crust?
Rock samples collected from around the world show that the chemical composition of the Earth's crust is not uniform, but certain elements are much more abundant than others (Figure 2-17). The elemental composition of the Earth's crust, oceans, and atmosphere are directly linked to the processes that formed the planet within the Solar System billions of years ago (as discussed in Chapter 1).
Note that oxygen and silicon are the two most abundant elements in Earth's crust. Therefore, compounds that contain some silicon and oxygen are the most abundant in rocks in the Earth's crust. These compounds occur as common silicate minerals that occur in abundance wherever rocks of certain origins occur on the surface. Ten of the common minerals (left and center columns in Figure 2-18) are silicate minerals.
|
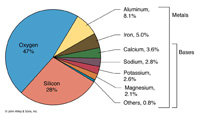
Fig. 2-17. Elemental composition of the Earth's crust. |
2.12
What are minerals? What are their significance?
A mineral is a naturally occurring, inorganic (never living) solid with a definite internal arrangement of atoms (crystal structure) and has a have a chemical formula that only varies over a limited range that does not alter the crystal structure. The crystal structure of a mineral has a definite internal arrangement of atoms (discussed below).
Currently there are about 4,000 known minerals of different chemical composition and internal atomic crystal arrangements (discussed below). However, slightly more than a dozen are considered common minerals because of their abundance on the earth surface. Figure 2-18 shows the most common rock-forming minerals.
In contrast, minerals considered gems are, mostly, exceedingly rare. Most minerals are chemical compounds consisting of two or more elements, however, some elements naturally occur in mineral form including gold, copper, platinum, sulfur, and iron.
What is the difference between a rock and a mineral?
A rock is a relatively hard, naturally formed aggregate of mineral matter or petrified matter. Rocks are mixtures and may consist of one or more minerals, but may include organic matter and other non-mineral substances, such as gases and water. Rocks are what makes up the materials of the solid Earth and other rocky planets and moons in the Solar System. The word stone is another common term used to describe rock.
Rocks consist of one or more minerals. Figure 2-19 shows how minerals can be combined to form different kinds of rocks that form under different environmental conditions.
The mineral composition of a rock reflects the physical environment and geologic history where a rock formed. Rock form in a variety of geologic setting ranging from locations on or near the surface, deep underground, or even in outer space. Most of the rocks we see on the surface of our planet formed by processes that happened long ago. However, we can see these processes that form rocks actively taking place in many places today. Rapid rock formation can be seen happening such as lava cooling from a volcanic eruption in places like Hawaii or Iceland. However, most rocks we see around us form very slowly in settings that may not be visible on the land's surface. Slow processes creating rocks can be inferred by observing reefs growing and accumulating in the oceans, or sediments being carried by flowing water in streams or moved by waves crashing on beaches. We can see sediments being deposited, but we cannot see them turning into stone because the process may take hundreds, thousands, or even millions of years, involving a variety of post-depositional processes.
The mineral composition of a rock reflects the physical environment and geologic history where a rock formed.
|
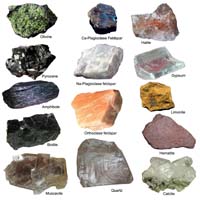
Fig. 2-18. Common rock-forming minerals are the most abundant minerals found on our planet Earth.
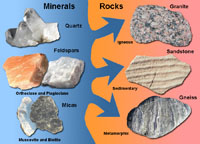
Fig. 2-19. Combinations of common minerals occur in different kinds of rocks. The kind of rock depends on the geologic setting where they form: igneous, sedimentary, or metamorphic (discussed below). |
2.13
"Every Rock Has A Story"
Rocks are composed of minerals—naturally occurring, crystalline chemical compounds. Rocks are composed of particles ranging from microscopic grains to full-sized crystals. These crystal grains can consist of different kinds of minerals that display many different identifiable physical characteristics. However, rocks may also contain compounds that are not minerals, such as organic compounds or residues that may not have distinct mineral characteristics (such as a definite crystal structure and composition). For instance, coal is a rock that is composed of materials of organic origin.
It is conceptually important that each rock (and its mineral components) has an origin in unique concepts of place, time, and physical and chemical conditions. Once rocks form, they are subject to change. These changes may be rapid (such as in a volcanic explosion) or taking place gradually over hundreds, millions, or billions of years. Some rocks have move great distances from their place of origin—both at the surface or to deep within the Earth's crust below. Trying to explain the what, how, and when of a rock's journey is fundamental to explaining why rocks are significant to resolving questions about our Earth's history and conditions within the physical environments where we live.
|
Fig. 2-20. Gypsum crystals from a cavern wall in Jewel Cave, South Dakota. |
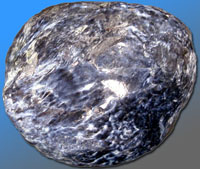
Fig. 2-21. Serpentinite, the state rock of California, is a metamorphic rock composed of serpentine minerals (of which there are many varieties). |
2.14
The Rock Cycle
The rock cycle is a graphic and conceptual model used to illustrate common rocks and earth materials and the processes that form or change in the Earth’s crust over time (Figure 2-22). The rock cycle depicts the series of events in which a rock of one type is converted to one or more other types and then back to the original type (see the general classification of earth materials below). Both products (rocks and sediments) and processes (such as melting, cooling, erosion, deposition, metamorphism, remelting) are part of this idealized cycle. The passage of geologic time is the essential component, although some processes are much faster than others. Note that all these types of processes are taking place simultaneously, but at different locations on and within the crust. It is important to note that rock cycle processes occur on other rocky planets or moons but rates may vary due to the presence (or lack of) atmospheric gases or fluids (including water) or availability of heat enough to melt rocks. The rock cycle and basic geologic principles are discussed in Chapter 3. |
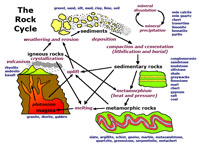
Fig. 2-22. The Rock Cycle is a conceptual model that portrays processes and products changing over time |
2.15
Rocks are classified into three general types based on their geologic origin.
Rocks are classified into three general groups: igneous, sedimentary, and metamorphic.
The term igneous applies to rocks or minerals that solidified (crystallized) from molten or partly molten material—referring to magma (molten material underground), or lava (molten material on the surface of a volcano) (Figure 2-23). The word igneous also applies to the processes related to the formation of such rocks. (Igneous rocks and volcanism are the focus of Chapter 7).
The term sedimentary applies to materials consisting of sediments or formed by deposition. The word sedimentary applies to both the processes and the products of deposition. Sedimentary rocks form from sediments (Figures 2-24 and 2-25). (Sediments are discussed in Chapter 8; Sedimentary rocks and processes are the focus of Chapter 9.)
The term metamorphic pertains to the process of metamorphism or to its results. Metamorphism is the chemical, mineralogical, and structural adjustment of solid rocks to changing physical and chemical conditions imposed at depth below the surface and below surficial zones where sedimentary processes take place (Figure 2-26). (Metamorphic rocks and processes are the focus of Chapter 10.)
In summary, characteristics of rocks include:
1) Rocks may be composed of a single type of mineral, or may be a mixture of minerals.
2) Rocks may also have organic residue (non-mineral) components.
3) Rocks preserve evidence of the physical environments in which they form. |
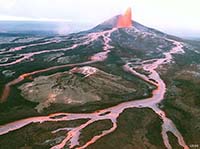
Fig. 2-23. Igneous rocks form from the cooling and solidification of molten material, such as lava erupting from a volcano. This view is of Pu'u'o'o Vocano in Hawaii Volcanoes National Park. |
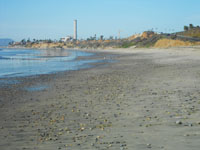
Fig. 2-24. Sediments form from disintegration of other rocks through processes of weathering and erosion to sites where they are deposited. This view is North Carlsbad State Beach, California |
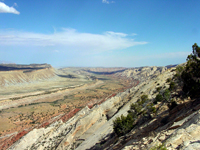
Fig. 2-25. Sedimentary rocks form from the consolidation and solidification of sediments where they are deposited and preserved. This view is in Capitol Reef National Park, Utah. |
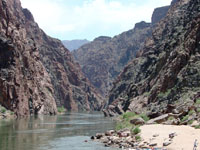
Fig. 2-26. Metamorphic rocks typically form under great heat and pressure deep underground, such as in the roots of actively forming mountain ranges. This view is in the Grand Canyon, Arizona |
|
2.16
What is a crystal?
A crystal is a solid substance with a homogeneous composition having an internal geometrically symmetrical atomic structure. Crystals can have external plane faces in symmetrical form that can be expressed on crystal surfaces. This is explained in more detail below.
What is Crystallography? Crystallography is the branch of science that studies the physical and chemical properties of crystals. Crystallographic studies typically focus on the internal arrangement of atoms within the crystalline structure of a gem, mineral, or chemical substance with an internal crystalline character. Most pure physical-chemical substances have at least one form of crystalline structure. Many substances have multiple crystalline forms related to the physical and environmental conditions in which they form.
|
Most gems are minerals that have unique arrangement of atoms in a crystal structure. The physical and chemical properties of the elements within the crystal structure give gems their unique properties!From the perspective of a gemologist (a person who studies, prepares, or sells gems) a mineral is an exciting thing! Most gems are minerals (Figure 2-27). Even common minerals in their natural form can be quite beautiful, valuable, and artistic if not used in jewelry (such as the gypsum crystals in Figure 2-20).
|
Fig. 2-27. Classic gems.
Natural gemstones are minerals. Most gems have unique identifying physical characteristics, such as color, hardness, and crystal structure. (However, some things considered gems, such as amber, pearls, obsidian and natural glass are not minerals.)
|
Learn more about gems and minerals at the Gemological Institute of America
If you live in the San Diego area, consider taking a guided tour at the Gemological Institute of America (GIA) in Carlsbad, CA. The GIA offers tours of their facilities where students and professionals are taught how to identify, evaluate, and work with gems and precious stones. The main building at GIA Headquarters has hallways lined with exhibits filled with exceptional examples of gems and provide discussions about their nature and occurrence. The GIA a large hall with special exhibits, and a room with one of the best mineral and gem-bearing rock exhibits in the world. The tour is free, but must be scheduled by reservation in advance. GIA is an excellent place to learn more about gems and minerals. Note that San Diego County has a long history of gem mining, and GIA provides a lot of information about historic mining operations with spectacular examples of local tourmaline, morganite, and other gem minerals. |
2.17
Crystalline vs. Non Crystalline Substances
As stated above, a crystal is a piece of a homogeneous solid substance having a naturally geometrically regular form with symmetrically arranged plane faces. Note that this geometric arrangement occurs on an atomic level (too small to see even with a powerful microscope), but this basic atomic arrangement repeats itself many trillions of times to form a single crystal grain.
A crystalline substance has the structure and form of a crystal or is composed of crystals. In our world there are many crystalline substances. To illustrate, let's start with salt (chemical formula - NaCl, or sodium chloride) or as geologists call it, the mineral halite. Salt usually precipitated from evaporating water without organic processes and is thus a
mineral. Halite is a very soft mineral because it's elements, sodium and chlorine are held together by ionic bonds.
Sugar (C6H12O6, sucrose) also forms crystals when precipitated from water, but because it is organic and therefore it is not a mineral. Sugar sold as rock candy has a mineral like appearance because it has a crystalline form, but it is not a mineral.
Very few things that are solid are not crystalline. However, because in our world much of what
we see is formed by life processes, most observed solids are not minerals. You will quickly
argue that rocks are all around us and that they are made of minerals, however in terms of variety
only about a dozen minerals (the rock forming minerals) are abundant, and in fact there is a great
deal more variety of organic solids around us than minerals. We rarely spend much time
observing minerals and really the average beginning student knows almost nothing about them
and their properties.
Figure 2-28 shows an organized mineral structure with an ordered arrangement of atoms (considered crystalline) and a disorganized substance without a crystal structure (considered non crystalline). Both can be solid, but a disorganized solid is called non-crystalline or amorphous. Both are held together by chemical bonds, but crystalline
solids have an ordered structure that fills space in 3 dimensions. With a crystalline structure you can predict where the next
atom can be found in the crystal structure.
Of the few inorganic, non-crystalline solids dealt with in gemology, glass is the most important. Glass is an amorphous substance that has no orderly arrangement of atoms (it is non crystalline). Glass is also a mixture of chemical compounds. Glass forms by rapid cooling of substances that have been melted to a liquid. There are natural
and man-made glasses. Man made glass is often used as a gem substitute, commonly as costume jewelry.
|
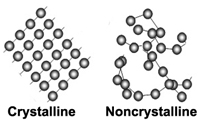
Fig. 2-28. Minerals are made up of atoms arranged in a crystalline structure. The crystals may range in size from on a microscopic scale to full-sized visible masses. Non crystalline (amorphous) substances (like glass) have no orderly arrangement of atoms. |
2.18
Atomic Structure of Crystals |
Minerals are chemical substances composed of atoms arranged in unique crystal structures.
A crystal structure describes a highly ordered repeatable arrangement of atoms. Note that there is an important difference between the chemical formula of a mineral and the molecular crystal structure of a mineral! A chemical formula is only a description of the elements that make up a pure chemical compound. Only when molecules are arranged in an orderly, repeatable symmetric pattern will it be considered a mineral. For instance, water (H2O) is not a mineral, but ice is!
Unit Cells In Crystals
A crystal structure can be thought of as an infinitely repeating array of three-dimensional boxes known as unit-cells. The unit cell is calculated from the smallest and simplest possible representation of molecules arranged to form a repeating crystal structure. Crystals consist of repeating unit cells ranging from the atomic level to consisting of many quadrillions of unit cells combined together in observably visible crystals (see example with halite below).
A simple way to illustrate the arrangement of atoms into a geometric crystal structure is to use marbles stacked in different ways (Figures 2-29 and 2-30); these forms shown ins how when marbles are stacked in different ways they can illustrated the simplest forms of different crystal arrangements. Figure 2-29 illustrates vertically stacked marbles, whereas Figure 2-30 shows marbles stacked in an offset arrangement that is the most tightest possible with spheres of uniform size. Figure 2-29 shows cubic and rectangular cuboids, and octagons (double pyramid) forms. Figure 2-30 shows hexagonal prism and pyramidal forms. In both illustrations, the marbles are the same size, only the stacking arrangement is different. Note the unit cell arrangement for each of the 4 forms. |
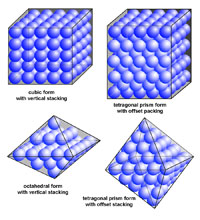
Fig. 2-29. Vertically stacked marbles illustrate atomic arrangement of crystal forms. Cubic, rectangular cuboids, and octahedral forms can form from the two arrangement of marbles. Note that a minimum of 8 marbles represents the minimum unit cell to create the larger vertical stacked and offset vertically stacked cubic structures. |
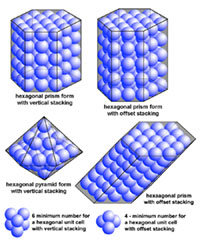
Fig. 2-30. The same arrangement of stacked, offset marbles can produce hexagonal crystal forms (prisms and pyramids). Add more layers of marbles (atoms) and the crystal grows larger.
Note only 6 marbles are needed for vertical hexagonal unit cell, and 4 marbles are needed for a offset-stacking hexagonal unit cell. |
2.19
Common and Important Minerals Illustrated
The discussions figures below illustrates the crystal structures of common or important minerals. Note that the arrangement of atoms in the mineral crystal structures illustrate below are magnified and expanded many millions of times from how they may appear on a molecular scale. Fortunately, scientists over the centuries have developed many tools for figuring out ways to indirectly see and interpret the microscopic crystal structure of minerals! Important rock forming minerals are illustrated below.
Halite (common table salt)
Figure 2-31 shows halite (or common table salt, NaCl) which consists of two elements sodium (Na) and chlorine (Cl) that when combined in a repeating arrangement in a crystalline structure (see Figure 2-32). Note that it would take 4 NaCl molecules (4 sodium atom and 4 chlorine atoms) to make of the minimum cubic unit cell representing the crystal structure of halite. The arrangement of atoms in a cubic structure of the mineral, halite, is repeatable whether on an atomic scale or a microscopic scale (as in table salt) or macroscopic (fist-sized chunk)(Figure 2-33). Halite crystals grow from precipitating from water and is manufactured worldwide by evaporating seawater (see Figure 2-34 as an example where and how it is done using evaporation ponds in arid settings). |
Why can't we directly see the internal crystal structure of solid substance? It is too small to see directly even with our most powerful microscopes!
Note that there are roughly about 2.4 x 1018 atoms in a single grain of salt! Written out, that number is: 2,400,000,000,000,000,000.
Fortunately, over the past centuries, mineralogists have found a variety of methods to indirectly determine the atomic structure of minerals and other substances using optics, x-rays, and other physical and chemical scanning methods.
|
|
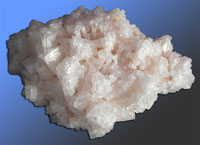 |
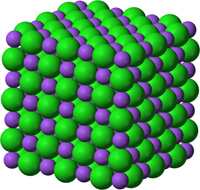 |
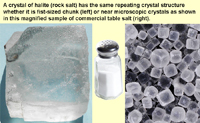 |
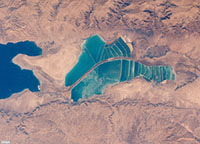 |
Fig. 2-31. The mineral halite is the raw material in the manufacture of table salt or, for melting ice on frozen walkways, rock salt. Most commercial salt comes from large underground mining operations. |
Fig. 2-32. Crystal structure of salt: the mineral halite
Chemical formula: NaCl (sodium chloride); Crystal form: cubic. The atoms are held together with ionic bonds. |
Fig. 2-33. Halite (salt) has the same cubic crystal shape no matter if the sample is fist-sized or ground up into table salt. Salt crystals (large or microscopic) all show 90º corner angles. |
Fig. 2-34. Halite is mined or is manufactured by concentrating sea water or salty water, as shown here in these evaporation ponds located near the Dead Sea. |
|
2.20
Fluorite
Figure 2-35 shows the crystalline structure of fluorite. Although the chemical formula of fluorite is CaF. However, it takes eight atoms of calcium (Ca) and sixteen atoms fluorine (F) are needed to make the minimum-sized unit cell of the crystal structure of mineral fluorite (Figure 2-36). Billions of unit cells are required to combine to make a single small crystal you can hold in your hand! The geometric arrangements of unit cells on an atomic scale determine how a crystal appears on a macroscopic (visible) scale (Figure 2-37). Because minerals have repeating geometric arrangement of atoms in crystal lattices, crystals can be fashioned into a variety of shapes that are compatible with the crystal structure. In the case of fluorite, which usually exists in cubic crystals, it can be split and shaped into octahedral shaped crystal specimens (commonly sold in rock shops)(see Figure 2-23). The arrangement of molecules within a crystal structure determines how a mineral crystal can be split and cut into geometric shapes, including shapes used in finished gemstones (as illustrated in Figures 2-38). It is important to note that in most cases, the shape of a fashioned gemstone is nothing like the shape of a natural mineral crystal shape as they appear in nature. A gemologist cutting gemstones will closely examine the crystal structure of a mineral before faceting it into a gemstone. |
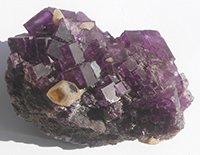 |
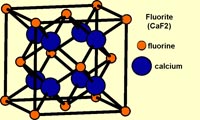 |
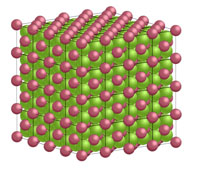 |
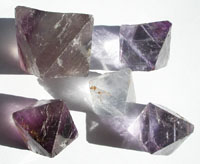 |
Fig. 2-35. Cubic crystal masses of the purple mineral fluorite. |
Fig. 2-36. Unit cell of the cubic crystal structure of the mineral fluorite
Chemical formula: CaF2 |
Fig. 2-37. Unit cells of the mineral fluorite combine to form an extended crystal lattice in three directions. |
Fig. 2-38. Although the crystal structure of fluorite is cubic, chunk of fluorite crystals can be split (faceted) along cleavage planes to form octahedral shaped crystals. |
|
2.21
Carbonate Minerals: Calcite, Aragonite, and Dolomite
Carbonate minerals have carbonate ions (-1CO3) within their mineral structure. In nature, most carbonate minerals form from the interactions of carbon dioxide and metals dissolved in water.
The mineral calcite is perhaps the most amazing mineral. It has many crystalline forms and can form in many geologic settings. It is also an exceeding important mineral resource - it is used in the manufacture of cement, and is used in some manner in the process of manufacturing of thousands of compounds used in industry, including the manufacture of steel and the production of medicines and food. Calcite consists of a crystalline structure composed of molecules of calcium carbonate (CaCO3). Basically, the calcium (Ca) comes from the Earth, and the CO3 comes from the atmosphere, and nearly all the CaCO3 is deposited by biological activity in the oceans and precipitated from water underground.
It is important to note that CaCO3 is a chemical formula only represents a single molecule. It takes many molecules of CaCO3 to make the unit cell of pure mineral calcite (see Figure 2-39). A pure specimen of calcite (CaCO3) would be perfectly clear form called Iceland spar (discussed below with Fig. 2-68). With pure calcite the unit cells will have 28 molecules of CaCO3, however, there can be a variety of other elements that can be substituted for a few of the calcium and carbon atoms with a unit cell, and it will keep the general crystal pattern of calcite. Elements including sodium, magnesium, iron, zinc, chromium, strontium, barium, and sulfur and can sneak into the structure of the unit cell and still maintain the general character of crystalline calcite. However, these differences can result in varieties calcite with some subtle differences in physical properties including color, crystal form, and special properties including fluorescence, phosphorescence, and thermoluminescence (discussed below). Calcite also doesn't fit the definition of a true mineral because it can also be of biological origin—a product of respiration, excretion, and precipitated or incorporated into the skeletal structures in plankton, microbial deposits, algal and coral reefs, and incorporated tissue of plants, invertebrate shells, and the shell of eggs.
The arrangement of unit cells can produce differently shaped crystals. For example, calcite can form several variation including dogtooth spar, nail-head spar, and other combined forms of these crystal varieties (see Figures 2-40 to 2-41). Note that each of the crystal forms in Figure 2-25 have a hexagonal shape. This variation of crystal shapes is related to the physical conditions of where the mineral formed. Also important to note is that calcite also has an internal molecular arrangement that has a rhombohedral crystal form. This is because there are weaker bonds within the crystal structure that allow crystals to split along cleavage plains. Figures 2-42 to 2-44 show how the molecular arrangement of atoms (Ca, C, and O) give rise to the crystal structure of calcite that allows it to be split along cleavage plains. When a large crystal of calcite is crushed, all the fragments, even down to a microscopic level, will display the rhombohedral cleavage shape pattern.
|
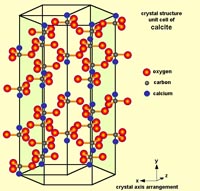 |
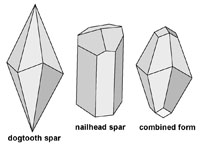 |
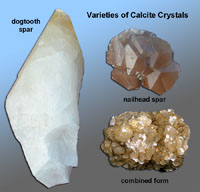 |
Fig. 2-39. Structure of the unit cell of the mineral calcite (calcium carbonate - chemical formula: CaCO3). It takes 28 molecules of CaCO3 to create the a single hexagonal shaped unit cell of calcite illustrated here on an atomic level. |
Fig. 2-40. Calcite crystals have a hexagonal crystal structure. The alignment of unit cells can form different crystal forms, all in hexagonal arrangement. Crystal forms of calcite include dogtooth spar, nail-head spar, and combined forms. |
Figure 2-41. Crystal forms of calcite: dogtooth spar, nail-head spar, and combined form. It takes many billions of unit cells combined to form visible crystals. Crystals like these form in open cavities underground where the crystals grow slowly over time. |
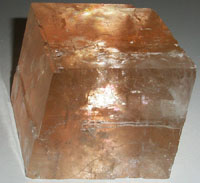 |
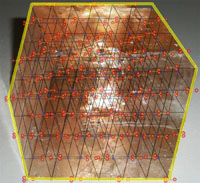 |
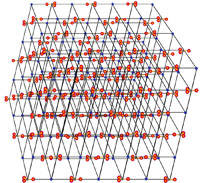 |
Figure 2-42. Calcite crystals can be split along mineral cleavage planes to form blocks with perfect rhombohedral shape. Note that this rhombohedral shape still retains its internal hexagonal crystal structure! |
Figure 2-43. Cleavage planes are naturally weak zones within a crystal structure. This image illustrates how molecules of calcium carbonate line up in repeating arrangement forming the rhombohedral shape. Note the hexagonal shape of the crystal block. |
Figure 2-44. Calcium carbonate molecules arrange in the rhombohedral structure of the mineral calcite. When a crystal of calcite is crushed it tends to split into many small pieces that retain a rhombohedral shape. These rhombs can range in size from microscopic to large blocks. |
|
2.22
Aragonite is another mineral composed of calcium carbonate (CaCO3) but has a different crystal structure and has different physical properties (Figure 2-45).
Dolomite is another carbonate mineral. Dolomite often forms from calcite by the substitution of a magnesium atom with a calcium atom (Figure 2-46). Calcite has a hexagonal crystal structure, whereas aragonite has an orthorhombic crystal structure (see crystal systems below).
Carbonate Rocks: Limestone and Dolostone
Calcite is the dominant mineral in the sedimentary rock called limestone. Over time, groundwater rich in dissolved magnesium can seep through limestone, gradually converting calcite to dolomite. As a result, ancient limestone rock formations often contain higher concentrations of dolomite than calcite. If a rock has more that 50 percent dolomite it is called dolostone. |
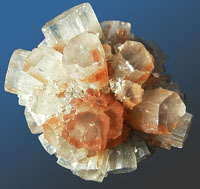
Figure 2-45. The mineral aragonite is also composed of calcium carbonate (CaCO3), but the molecules are in a different crystalline structural arrangement than calcite. |
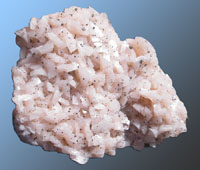
Figure 2-46. The mineral, dolomite, has a chemical formula of CaMg(CO3)2. It has a trigonal-rhombohedral crystal form. The pink color comes from traces of iron within the crystal structure. |
2.23
What Is Mineral Cleavage?
Mineral cleavage is the tendency of crystalline materials to split along definite crystallographic structural planes (or, for clarification, to break along smooth planes parallel to zones of weak bonding in crystalline substances). For instance, as illustrated above in Figures 2-42 to 2-44, calcium carbonate forms crystalline forms, calcite and aragonite. However, when a mineral sample of calcite is crushed, the crystals shatter along planes of weakness in the crystal lattice. In the case of calcite, the crystals break along 3 planes of weakness within the crystal structure, forming rhombohedral blocks. These cleavage planes are always at the same angles (in 3 directions, the x, y and z dimensional axes). The rhombohedral shape of the calcite crystal fragments are always the same, whether as a hand-size specimen or crystal fragments on a microscopic level. (The same is true for halite illustrated above in Figure 2-33, except the salt crystals are cubes instead of rhombs.) Mica minerals display exceptional mineral cleavage (including muscavite and biotite, shown in Figures 2-18 and 2-88).
|
|
2.24
Three factors play important roles in the physical properties of mineral:
1) the crystal structure,
2) character of chemical bonds within crystalline substances, and
3) the ability of substances to split along cleavage planes.
How the arrangement of atoms affect physical properties is easily illustrated with two carbon minerals, graphite and diamond. In their purist form, both mineral consist of the element carbon. In Figures 2-47 and 2-48, the lines between carbon atoms
represent chemical bonds. The structure of minerals and their bonding results in the
difference in the physical properties of the two minerals, for example hardness: graphite is one of the softest minerals (used in pencils) and diamonds are the hardest mineral (gems).
These
factors, particularly the hardness of a mineral and its tendency to split along cleavage planes, determine if and how a mineral specimen might be cut or faceted into a gemstone. |
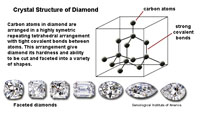 |
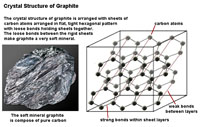 |
Figure 2-47. Crystal structure of the mineral diamond. |
Figure 2-48. Crystal structure of the mineral graphite. |
Although both diamond and graphite consist of the element carbon, the two minerals have very different crystal structure arrangements and associated physical properties. Besides differences in hardness, graphite can conduct electricity whereas diamonds cannot conduct electricity. |
|
2.25
How Many Crystal Shapes Are There?
Well over 4,000 different minerals have been identified occurring naturally in the world. There are probably many more. Hundreds of thousand of inorganic compounds are known (and patented) and perhaps billions of organic compounds exist (having carbon and hydrogen and other elements combined in complex molecules). However, with all the chemical compounds that are known, there are only a relatively low number of naturally occurring, common or important mineral compounds that are gems or have economic significance.
Figures 2-49 to 2-54 illustrate a classification of natural crystal forms and shapes (grouped within crystal systems). Minerals have characteristic crystal shapes that can be used to help identify them. |
Crystal Systems - Crystal Forms and Selected Crystal Shapes |
Figure 2-49.
Cubic and
isometric
crystal system
Crystal forms:
include cube, octahedron, dodecahedron, and other more complex forms. |
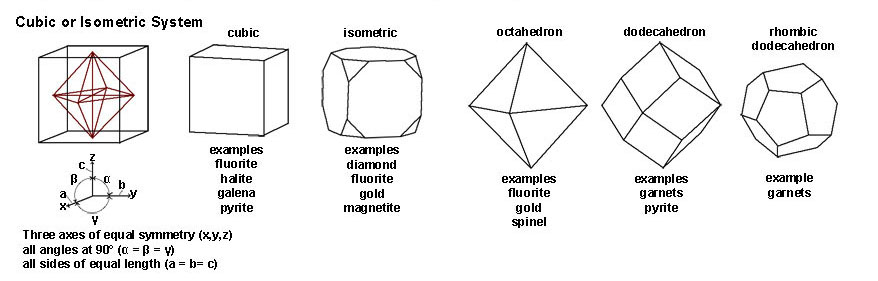 |
|
The Cubic or Isometric System include all crystal shapes that have symmetry axes in equal lengths in 3 directions (at 90º angles to each other). Common minerals that have a cubic/isometric crystal form include halite, fluorite, galena, pyrite, magnetite. Gem minerals include diamond, garnets, spinel, and gold. |
Figure 2-50.
Tetragonal
crystal system. "Rectanguloid" shapes, prisms, pyramids, and complex forms. |
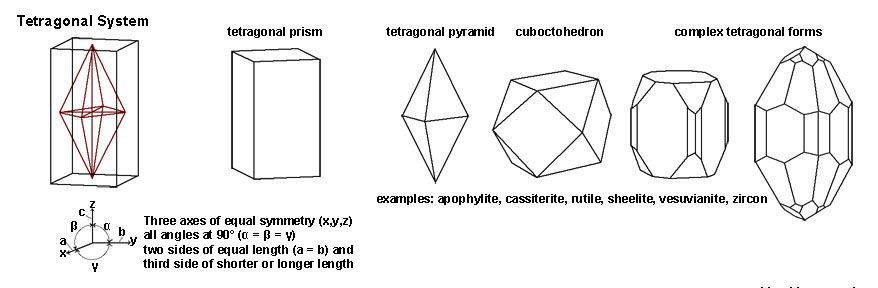 |
The Tetragonal System includes all crystal shapes that have three axes of symmetry all at right angles (90º) of each other. However, two sides of the crystal axes share equal length, whereas the length of the third axis is either shorter or longer than the other two. Some examples of minerals include apophylite, cassiterite, sheelite, and vesuvianite. Gems include zircon and rutile. |
Figure 2-51.
Hexagonal
crystal system: six-sided prism, pyramid-shaped, rhombohedral, and combined forms. Both calcite and quartz produce a variety of crystal shapes within the hexagonal or trigonal forms. |
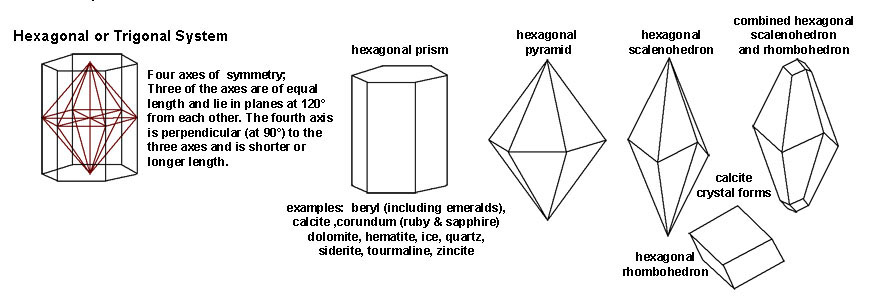 |
The Hexagonal or Trigonal System includes crystal shape that are hexagonal. Three of the crystal axes are of equal length and lie in planes that are 120º from each other. The fourth axis is perpendicular (90º) to the three axes and is either shorter or longer to the other axes. Minerals with hexagonal form include calcite, dolomite, hematite, ice, quartz, and siderite. Gem minerals include beryl (including emerald), corundum (including ruby and sapphires), quartz varieties (crystal, citrine, amethyst), and tourmaline. |
Figure 2-52. Orthorhombic
crystal system: prisms, pyramids, and combined forms. |
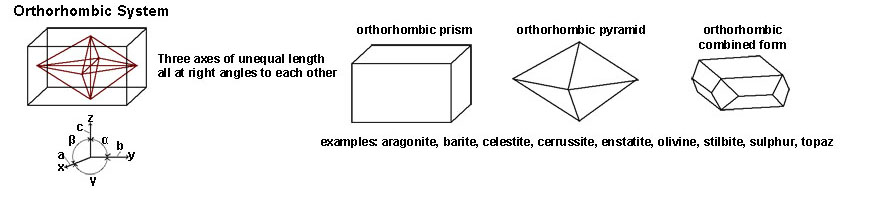 |
The Orthorhombic System includes crystal shapes that have three axes of equal length but all at right angles (90º) of each other. Minerals with orthorhombic forms include aragonite, barite, celestite, cerrussite, enstatite, olivine, stilbite and sulfur. Gem minerals include peridote (olivine) and topaz. |
Figure 2-53.
Monoclinic
crystal system |
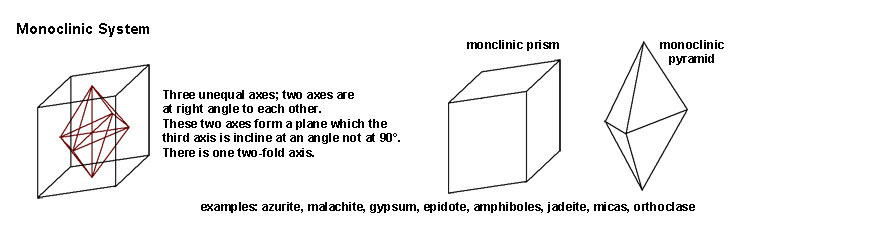 |
The Monoclinic System includes crystal forms that have three unequal axes; two of the axes are at right angles (90º) but the third axis is inclined at an angle not at 90º. There is one two-fold axis of symmetry. Mineral examples include azurite, malachite, gypsum, epidote, amphiboles, jadeite, micas, and orthoclase. |
Figure 2-54. Triclinic
crystal system |
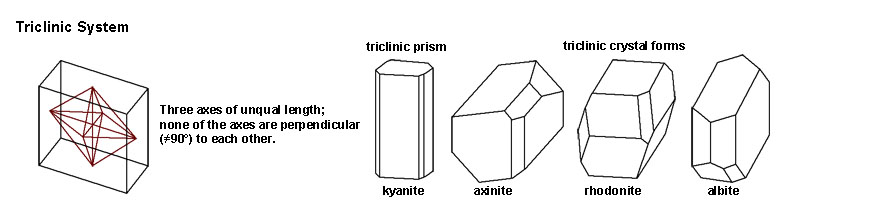
The Triclinic System includes crystal forms where the three axes are of unequal length, and one of the axes are perpendicular to each other. Mineral examples include kyanite, axinite, rhodonite, and albite. |
2.26
How can physical and chemical properties of minerals be used for their identification? |
All minerals have unique properties that aide in their identification. Some minerals have unique characteristics that have an appearance or physical properties that make them easy to identify. However, these identifying characteristics may not be easy to determine without more extensive testing. Fortunately, the most common minerals are fairly easy to identify by their general appearance or with simple tests for hardness, crystal form, color, magnetism, and streak (Streak means it leaves a colored line when scratched on a piece of tile or hard surface). Note that some tests can be destructive to mineral samples (such as measuring hardness, streak, malleability, elasticity, and testing with acid). In addition, tasting a mineral is definitely not recommend - some are actually poisonous! Washing your hands after handling unknown mineral samples is always recommended. |
Observable Characteristics and Tests for Identifying Minerals |
Easily Observable Characteristics |
Simple Tests Requiring Equipment |
crystal form
color
cleavage
luster (metallic, non-metallic)
diaphaneity (transparent, translucent or opaque)
double refraction
striations
feel
odor (smell)
taste
|
hardness
magnetism
streak
acidic reaction
specific gravity
malleability
elasticity
electrical resistivity fluorescence
phosphorescence
radioactivity
thermoluminescence |
|
2.27
Observable Properties of Minerals
The following physical properties can be used to identify a mineral through sensory observations or conducting simple tests. Equipment for such tests are typically available in science education departments or are available from commercial sources.
|
Easily observable physical characteristics (simple visual observations of the form and character of some minerals) are illustrated below. |
2.28
Crystal form—many minerals have unique and sometimes obvious crystal structures, however, crystal structure alone may not be enough to identify a mineral. For most samples used in mineral tests, crystal form may not be apparent or easily measurable.
|
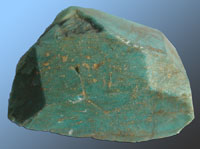 |
Fig. 2-55. Amazonite is a blue-green form of microcline feldspar. Samples of feldspars are fairly easy to find or purchase, and they typically have good crystal form (angles) for students to measure. |
2.29
Color—some minerals have very distinct colors, however, color is not a reliable indicator by itself. |
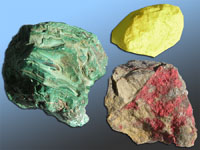 |
Fig. 2-56. Some minerals have obvious color associations. The combination of color with other mineral characteristics make the easy to identify: malachite (green), sulfur (yellow) and cinnabar (blood red). Problems arise with mineral samples are white or gray - there are dozens of minerals that have those neutral tones and make them difficult to easily identify without other tests. |
2.30
Cleavage—the tendency of a crystallized substance to split along definite crystalline planes, yielding smooth surfaces. Mica, feldspar, calcite, and selenite gypsum have good mineral cleavage. Flat, smooth, shiny and reflective surfaces on specimens may be either crystal surfaces and/or cleavage. |
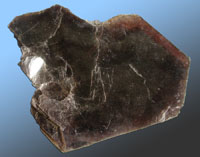 |
Fig. 2-57. Many minerals have cleavage planes that make them easy to identify, with micas (biotite is black mica, muscovite is silvery-white mica) being perhaps the most easy to recognize. Crushing irregularly shaped samples may demonstrate repeatable shapes associated with cleavage planes. Common minerals that easily display cleavage patterns when crushed or broken include calcite and feldspars. |
2.31
Striations—some mineral crystals have fine, narrowly-spaced lines on crystal surfaces. (Examples of minerals that may display striations include hornblende, pyrite and selenite (a crystalline form of gypsum).
|
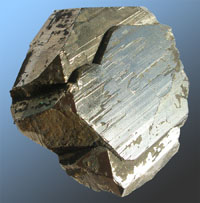 |
Fig. 2-58. Mineral crystals that grow in open cavities sometime display striations that are parallel to the crystal axes within the mineral's crystal structure. This sample shows a pyrite crystal with obvious striations. Note that striations may not occur on all all examples of a mineral. For example the cube-shaped pyrite specimen shown in Figure 2-44 does not display striations. |
2.32
Luster—the description of the quality and intensity (sheen or shine) of light reflected off of a mineral, particularly a reflective appearance of the exterior of crystal surfaces and cleavage planes. There are many kinds of luster:
- Metallic means having the appearance of polished metal. Native copper, gold, silver, and platinum have metallic luster on polished surfaces. Metalloid minerals including galena and pyrite have high metallic luster (Figure 2-59).
- Adamantine means having the hardness or luster of a diamond. Clear diamond is a highly radiant in bright light. Other minerals with high radiance include cubic zirconium, and "Herkimer diamond" (a unique variety of very clear quartz crystal). Most of the gems in Figure 2-27 display an adamantine luster.
- Chatoyancy is the character of having a fibrous texture as seen in
tiger eye. Tiger eye has fibers embedded in quartz and has a strong chatoyancy (Figure 2-60). Other
minerals such as tourmaline and cats eye (chrysoberyl), or chrysotile also show this.
- Schiller is luster property best seen in
labradorite feldspar that varies in color as the mineral is moved and looks like the wings of some
iridescent butterflies (Figure 2-61). Labradorite makes an attractive building material and semiprecious stone.
Schiller is also seen in some gems such as moonstone.
- Pearly luster as seen in variety of gypsum (called satin spar)(Figure 2-62). Ulexite is sometimes called the "TV stone" because of it's optical fiber light transmission properties (see Figure 2-55 below).
- Greasy luster as in some chalcedony, a type of
microcrystalline (also called cryptocrystalline) variety of the mineral quartz (Figure 2-63).
- Vitreous luster as seen in broken glass. On fresh, broken surfaces it has a conchoidal fracture pattern, like broken glass. Quartz crystals have a vitreous luster on broken surfaces. Obsidian (a natural glass [rock]) also has a vitreous luster (Figure 2-64).
- Resinous luster as seen in amber. Note that amber is a fossilized tree resin; not a mineral (Figure 2-65).
- Earthy means having a dull or matte like appearance, like the texture of a terracotta flower pot. Minerals like hematite and limonite that typically consist of very fine microscopic crystals have an "earthy" (dirt-like) texture (see cinnabar [red], sulfur [yellow], and malachite [green] in Figure 2-56).
|
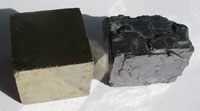 |
Fig. 2-59. Pyrite (left) and galena (right) have a metallic luster. |
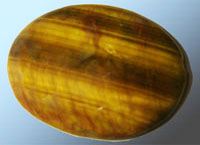 |
Fig. 2-60. Tiger eye (a variety of quartz) displays chatoyancy luster. |
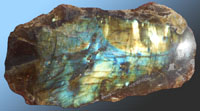 |
Fig. 2-61. Labradorite (a variety of feldspar) displays a schiller luster. |
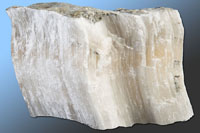 |
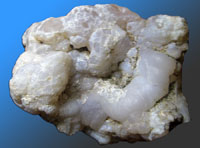 |
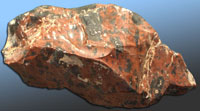 |
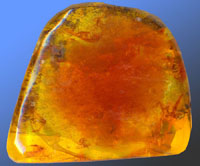 |
Fig. 2-62. Satin spar, a variety of the mineral gypsum displays a pearly luster. |
Fig. 2-63. Chalcedony, a variety of the mineral quartz, has a greasy luster. |
Fig. 2-64. Obsidian, a natural glass, has a vitreous luster. It is a rock, not a mineral! |
Fig. 2-65. Amber has a resinous luster. It is actually fossil tree resin!
It is a rock, not a mineral! |
|
2.33
Optical Properties of Minerals
Transparency—or more correctly, diaphaneity, is an evaluation of how light passes through a mineral, with general descriptions of being transparent (meaning clear enough for an object to be seen through a sample); translucent (a substance transmit light but it is dispersed or cloudy in appearance), or opaque (a substance will not transmit light). Few common minerals are transparent. Quartz and calcite can have high transparency (see Figures 2-66 and 2-68). Common milky quartz is typically translucent (light passes through but is diffuse, Figure 2-67). |
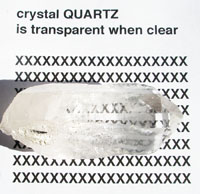
Fig. 2-66. Crystals of pure quartz are transparent like glass when clear. |
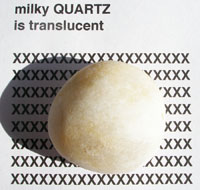
Fig. 2-67. Milky quartz is translucent (cloudy, but allows light to be transmitted). |
2.34
Double refraction—light passing through clear calcite (a variety called Iceland spar) will transmit a double image. Clear calcite can split a laser beam into two separate beams. Figure 2-68 shows a piece of Iceland spar causing the X pattern of the underlying paper to be doubled on itself. |
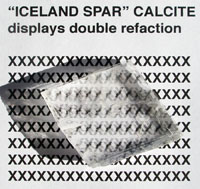
Fig. 2-68. Clear calcite displays double refraction. |
Fiber optic properties—a notable example is ulexite, a soft borate mineral moves images from one side of a cut sample to the other side with a cut surface. Figure 2-69 shows the X pattern on the underlying piece of paper transmitted to the surface of the ulexite mineral sample. |
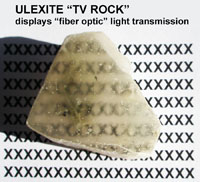
Fig. 2-69. Ulexite (also called "TV Rock") shows fiber-optic like properties. |
2.35
Non-Visual Sensory Characteristics of Minerals |
Feel—The feel of a rock is not a reliable method of testing minerals, however certain minerals have textures like "soft, silky, satin, smooth, hard, heavy or light" - but these characteristics are poorly definable as a reliable means for identifying minerals.
Odor—few minerals have an odor. Sulfur-bearing minerals may put off a rotten-egg like smell. Many rocks of sedimentary origin have the smell of petroleum.
Taste—halite tastes like salt (because it is NaCl). (Note that tasting minerals and rocks is generally not recommended! Some minerals can be quite poisonous.) |
2.36
Simple Tests For Identifying Minerals |
Minerals have a variety of physical and chemicals properties that can be evaluated using simple tests. The following tests are simple determinations using common laboratory equipment and supplies. Note that some of these are destructive to samples being tested! |
2.37
Hardness—minerals have different durability properties. Depending on mineral chemistry and crystal structure, minerals have varying degrees of hardness. Simple tests of scratching mineral samples with items or material of known hardness can give a general range of hardness of a specimen. Note that minerals held together by ionic bonds (like halite, gypsum, and calcite) tend to be softer than minerals
Mohs Hardness Scale is a list of hardness of common minerals (Figure 2-70) used in mineral testing laboratory exercises. Many gemstone have higher hardness. Diamonds are the hardest mineral, having a Mohs scale hardness of 10.
Note that testing the hardness of minerals may be destructive to samples! |
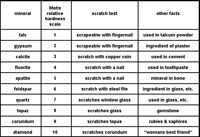
Fig. 70. Mohs Hardness Scale |
2.38
Magnetism—iron (the natural mineral iron in crystalline form) and magnetite (Fe3O4) are common magnetic rocks, iron-rich meteorites are also magnetic. Figure 2-71 is sample of Diablo Canyon (Arizona) iron meteorite that is highly magnetic.
Many minerals rich in iron are partly magnetic and display measurable magnetic susceptibility that can be useful for geophysical exploration. Large bodies of rock containing iron-rich minerals can be remotely detected below the earth surface, and may be useful for detecting hidden faults, water-filled sedimentary basins, or potentially economically valuable mineral resource deposits. Magnetic susceptibility measurement are used in regional geophysical mapping. |
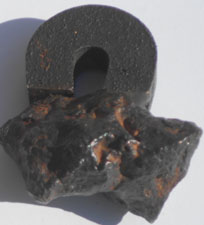 |
Fig. 2-71. Magnets stick strongly to some iron minerals, such as native iron (naturally pure iron in mineral form) and the common mineral, magnetite. Other minerals show weak magnetic attraction including iron-rich metallic and metalloid minerals: hematite, goethite, chromite, franklinite, pyrrhotite, and siderite. Shown here, a magnet sticks strongly to a meteorite composed of the metallic iron-nickel mineral crystals (kamacite and taenite). |
Common Rock-Forming Iron Minerals (Figures 2-72 and 2-73)
Hematite—A reddish, steel gray, or black mineral consisting of ferric oxide (Fe2O3).
Limonite—An amorphous orange to brownish mineral consisting of a mixture of hydrated ferric oxides, important as an iron ore. Rust on iron vehicles is essentially limonite.
Magnetite—a gray-black magnetic mineral that consists of iron oxide (Fe3O4) and is an important form of iron ore. Magnetite is highly magnetic.
Pyrite—a brass-colored mineral, FeS2, occurring widely and used as an iron ore and in producing sulfur dioxide for sulfuric acid. Also called fool's gold, iron pyrites. |
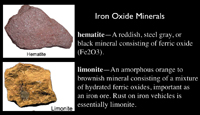
Fig. 2-72. Iron minerals: Hematite and Limonite |
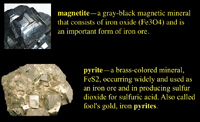
Fig. 2-73. Iron minerals: Magnetite and Pyrite |
2.39
Specific gravity—a measure of the density of a mineral. Specific gravity is the ratio of the density of a substance to the density of water. Tests for specific gravity require some laboratory equipment. Specific gravity is a measure of weight with a known volume (Figure 2-74). |
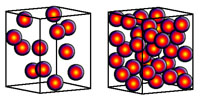 |
Fig. 2-74. Two equal size cubes with dots representing atoms. The box on the left has fewer atoms in the same amount of space as the second box. The second box would therefore be denser than the first box. In addition, some elements are much denser than other because of their molecular weight. For instance, compare pyrite and galena in Figure 2-59. Both samples are about the same volume, but the galena sample (PbS) is much heavier (denser) than the pyrite (FeS2). This is because lead (Pb) is a heavier atom then iron (Fe), and the atom crystal structure is more densely packed in galena. |
2.40
Streak—soft minerals may leave a streak of color on a piece of tile. Hematite makes a red streak, pyrite is brown, magnetite is black, etc. Be aware that streak tests can be destructive to mineral samples. The mineral graphite (used in pencils) leaves a black streak! |
2.41
Fluorescence—some minerals glow colors under a blacklight including some fluorite, calcite, and zinc minerals. Different minerals glow brightly (fluoresce) under different wavelength of ultraviolet light, sometimes in different colors under different wavelengths. The crystal structures of fluorescent minerals allow ultraviolet energy to be absorbed and the energy is released in a visible color wavelength (see Figure 2-60). Most rocks and minerals are not fluorescent. Only a few common minerals will glow under common blacklight that releases long-wave ultraviolet light. Many others will glow under short-wave ultraviolet lamps (that are potentially hazardous to use improperly).
Phosphorescence—some minerals absorb light energy and release light when the light is turned off. Some varieties of calcite, zinc minerals, and minerals rich in phosphorus sometimes display phosphorescence. Phosphorescence is only observable in a very dark setting - very shortly after energy source (visible light, or better, ultraviolet light) is shut off. In most cases, the phosphorescent glow ends quickly. Some phosphate-rich calcite and zinc minerals can glow for quite a some time after being exposed to a light source, with brightness decaying slowly over time. |
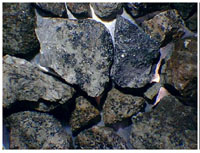 |
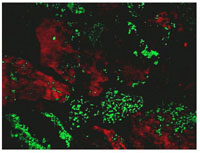 |
normal light
|
short-wave ultraviolet light
|
Fig. 2-75. Fluorescent minerals from Franklin, New Jersey. Under normal light (left) and under short-wave UV light (right): calcite glows red, and wilmenite and other zinc-bearing minerals glow green. Franklin, New Jersey is often called the "Fluorescent Mineral Capitol of the World" and is home to the Franklin Mineral Museum - an amazing place to visit! |
2.42
Thermoluminescence—some minerals will glow in colors when heated, similar to a hot burner on a stove or an object held under a torch flame. Note that heating gems and minerals samples can (and probably will) alter or destroy them.
|
2.43
Radioactivity— Radioactive elements that occur in rocks and minerals include potassium, thorium, radium, and uranium. and may display measurable radioactivity. Most mineral samples do not have measurable levels of radioactivity. However, many older collections in science departments may have radioactive mineral samples, and these should be clearly identified and not handled. Radiation, like magnetism and gravity, are used in geophysical mapping and resource exploration. Granitic rocks tend to be slightly more radioactive than other rocks having trace concentrations of uranium or thorium. Fossil wood from the Colorado Plateau region can sometimes be radioactive. It is advisable not to collect radioactive material because of the potential health risks. If collected, they should be clearly marked and stored in appropriate containers. They may be illegal to own or transported. |
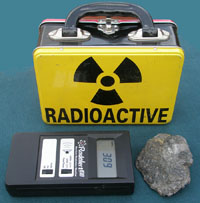 |
Figure 2-76. A geiger counter us used to measure materials for radioactivity. The sample shown here is a piece of gold ore from the Witwatersrand Gold Mine in South Africa. The gold is mixed in with uranium-bearing minerals and quartz. Many locations where gold occurs there may be other heavy elements, including uranium. |
2.44
Acidic reaction—Calcite fizzes when exposed to mild acid, releasing carbon dioxide gas. Dolomite will fizz in hot acid.
Note that all minerals are chemicals that can react to chemical agents, altering or destroying them. Whereas gemstones are typically durable, the can be susceptible to chemicals added to cleaning fluids. Iron-bearing mineral will react to oxidizing compounds like bleach. Be sure to check on appropriate cleaning agents before cleaning gemstones or gem-bearing jewelry
|
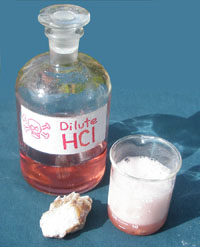 |
Figure 2-77. Some minerals will react to exposure to acid. Calcite [CaCO3] fizzes when exposed to hydrochloric acid (HCl) or vinegar (acetic acid), , releasing carbon dioxide gas.. Dolomite [CaMg(CO3)2] will fizz only in hot acid. Note that acid will not only destroy mineral samples but can also ruin clothes! |
2.45
Malleability—metals like gold, copper, iron, and silver is able to be hammered into objects.
Elasticity—soft minerals may be bendable (like mica); most minerals fracture or shatter when put under stress or shock.
|
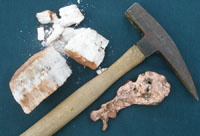 |
Figure 2-78. Whereas it is sometime fun to smash things, it is not really a useful means of testing minerals. |
2.46
Electrical resistivity—all native metals (gold, copper, silver) and many metalloid (metal-bearing) minerals will conduct electricity. Most metal ore minerals will conduct electricity. Common examples include iron ores: hematite, magnetite, pyrite, chalcopyrite, bornite, galena. Minerals with a metallic luster will conduct electricity. Conversely, non-metallic minerals will not conduct electricity. Quartz and calcite will not conduct electricity.
|
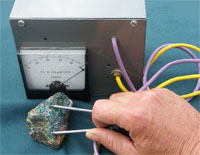 |
Figure 2-79. A simple electrical resistivity measuring device, shown here, has a battery, a micro-ampere meter, and wires attached to electrodes (nails). This test shows that a sample of bornite (copper "peacock ore") conducts electricity quite well. Parts of a flashlight can be used to make an electrical conductivity testing device. |
2.47
Understanding the nature of silicate minerals is important!
Silicate minerals are the dominant group of minerals that make up the rocky crusts of the Earth, Moon, and other stony planets (Mercury, Venus, Mars, and many other moons and asteroids within the Solar System).
Silicate minerals chemically consist of compounds that contain the geometric arrangement of silicon-oxide tetrahedrons contained within simple to complex crystalline structures. Other elements combine with the silicon-oxide to form many different minerals with unique physical properties.
Mafic vs. Felsic Minerals (and Rocks)
The Earth's crust and mantle are dominantly composed of silicate minerals and are commonly associated with varieties of igneous and metamorphic rocks formed in specific geologic settings (and are important to discussion about plate tectonics, discussed in Chapter 5). Common silicate minerals (and the rocks they form) are grouped into two general classes: mafic and felsic.
The term mafic refers to silicate minerals (and the rocks they form) that are enriched in the metals of magnesium and iron. Mafic materials (rocks and minerals) tend to be dark colored (Figure 2-80). Mafic minerals include varieties of olivine, pyroxene, and amphibole (illustrated below). Note that there are several varieties of each mafic mineral depending on other elements present in their crystal structures. Mafic rocks are commonly associated with rocks formed on ocean basin settings and are associated with very hot magmas derived from sources in the Earth's mantle.
In contrast, the term felsic (named after feldspar) are minerals or rocks rich in silica and aluminum relative to other metals. Felsic minerals (and the rocks they form) tend to be light colored (Figure 2-81). Common felsic minerals include quartz, certain feldspars (including orthoclase, and the sodium-rich plagioclase) and muscovite mica (see below). Felsic rocks are generally associated with rocks formed in continental settings.
Molten material that form felsic rocks and minerals typical comes from the melting (or remelting) of preexisting materials and are associated with magma (and lava) that is not nearly as hot as molten material associated with mafic sources.
|
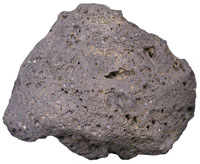
Fig. 2-80. Basalt is a dark colored igneous rock composed of mafic minerals. Basalt is the dominant rock found under ocean basins and exposed in places like Hawaii.
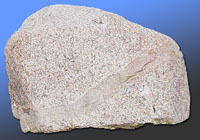
Fig. 2-81. Granite is an igneous rock made up of light-colored felsic minerals, mostly quartz and varieties of feldspar minerals. Granite is found in abundance in the core of continental regions. |
2.48
Crystal Structures of Common Silicate Minerals
Silicate minerals are the dominant group of minerals that make up the rocky crusts of the Earth, Moon, and other stony planets (Mercury, Venus, Mars, and many other moons and asteroids within the Solar System. Silicate minerals chemically consist of compounds that contain the geometric arrangement of silicon-oxide tetrahedrons contained within simple to complex crystalline structures (Figures 2-82 and 2-83). Silicon-oxide tetrahedrons combine to form chains, sheets, and other complex crystal arrangements. Other elements combine with the silicon-oxide tetrahedrons to form many different minerals with unique physical properties.
|
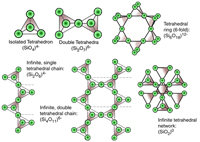 |
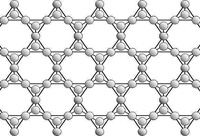 |
Fig. 2-82. Basic crystalline structure of common silicate minerals. |
Fig. 2-83. Structure structure of sheet silicates (including micas and clay minerals). |
2.49
Common Silicate Minerals |
Felsic Silicate Minerals |
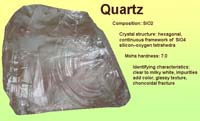
Fig. 2-84. Quartz
Fig. 2-85. Various forms of quartz found in mineral, sediment, and rock forms. |
Quartz
Common quartz is a hard colorless or white mineral consisting of silicon dioxide (silica-SiO2), found widely in igneous, metamorphic, and sedimentary rocks (Figure 2-84).
Quartz is a felsic mineral. Pure silica forms clear quartz crystals in unconfined spaces, such as geodes or open fissures in rock. Impurities and inclusion of traces of other element in quartz's crystalline structure add color and produce varieties of semiprecious gem varieties including amethyst, citrine, rose quartz, and smoky quartz, and many other forms (Figures 2-85 and 2-86). Microcrystalline varieties of sedimentary rock composed dominantly of quartz include chert, jasper, flint, agate, and chalcedony. |
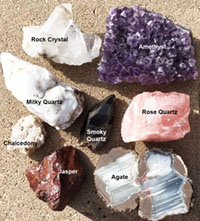
Fig. 2-86. Varieties of quartz in crystalline and microcrystalline forms. |
Figure 2-85. Varieties of quartz (mineral forms; top left to bottom right): quartz crystal (clear), amethyst (purple), milky quartz (white), smoky quartz (black), rose quartz (pink); microcrystalline varieties: chalcedony (white-gray), jasper (red), agate (multy-colored). |
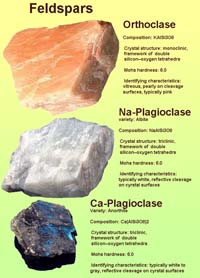
Fig. 2-87. Feldspars |
Feldspars
Feldspars are an abundant rock-forming group of minerals typically occurring as colorless or pale-colored crystals (Figures 2-87 and 2-88). Feldspars are aluminosilicate minerals with varieties:
Orthoclase or K-spar—a variety of feldspar that rich in potassium (KAlSi3O8),
Plagioclase—varieties of feldspar rich in feldspar which include sodium-rich Albite (NaAlSi3O8), and calcium-rich Anorthite (CaAlO2SiO2O8).
There are many other varieties of feldspars with variable compositions and appearances.
Orthoclase and sodium-rich plagioclase is associated with felsic rocks. In contrast, calcium-rich plagioclase forms at higher temperatures than sodium-rich plagioclase and is commonly mixed in with mafic minerals in mafic rocks. |
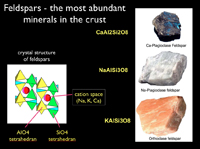
Fig. 2-88. Crystal structure of feldspars are combinations of silicon-oxide and aluminum-oxide tetrahedrons with elements of sodium, calcium, potassium and sometimes traces of other elements. |
Mafic Silicate Minerals |
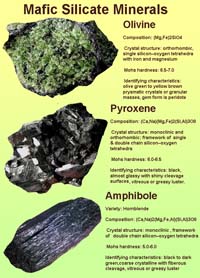
Fig. 2-89. Mafic minerals |
Mafic silicate minerals are rich in magnesium and iron. The word mafic is used to describe rocks containing a group of dark-colored, mainly ferromagnesian minerals (rich in iron and magnesium). Mafic rocks are common in the Earth's crust under the ocean basins and are exposed in the volcanoes of Hawaii and Iceland. Mafic and ultramafic rock include minerals such as pyroxene, olivine, and amphibole (and many other minerals).
olivine—a mineral silicate of iron and magnesium, principally (Mg,Fe)2SiO4, found in igneous and metamorphic rocks occurring in basalt, peridotite, and other basic igneous rocks.
pyroxene—Any of a large class of rock-forming silicate minerals, generally containing containing two metallic oxides combining magnesium, iron, calcium, sodium, or aluminum and typically occurring as prismatic crystals.
amphibole—Any of a class of rock-forming silicate or aluminosilicate minerals typically occurring as fibrous or columnar crystals consisting of hydrated double silicate minerals, such as hornblende, containing various combinations of sodium, calcium, magnesium, iron, and aluminum. |
2.50
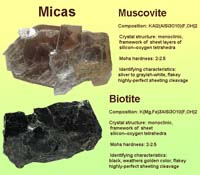
Fig. 2-90. Mica minerals
|
Micas And Clay Minerals
Micas and clay minerals are silicate minerals that have a sheet-like crystal arrangement that allow them to cleave into thin sheets (Figures 2-90 and 2-91).
Common micas include:
biotite—a common rock-forming mineral occurring in black, dark-brown, or dark -green sheets and flakes: an important constituent of igneous and metamorphic rocks. Biotite is a mafic variety of mica.
muscovite—a silver-gray form of mica (platy sheet silicate mineral) occurring in many igneous and metamorphic rocks. Muscovite is a felsic variety of mica. |
Fig. 2-91. Mica minerals easily peel into thin sheets that are quite flexible. This is related to their sheet-like crystal structure. There are a variety of different minerals with sheet-like crystal structures. |
Clay Minerals
Clay minerals are any of a group of minerals that occur as microscopic sheet-like or fibrous crystals in clay (Figure 2-92). Clay minerals are a primary component of many soils and form from the weathering decay of other silicate and aluminum-rich minerals, such as feldspars, micas, and other mafic minerals. Like micas illustrated above, clay minerals have sheet framework crystals.
Clay minerals form from the chemical breakdown of other silicate minerals that are not stable in wet surface conditions. Clays themselves are very stable in the surface environment. However, clay minerals will gradually convert back to other silicate minerals when subjected to heat and pressure associated with deep burial and metamorphism. Clay minerals are a major component of most kinds of soils (discussed in Chapter 8). |
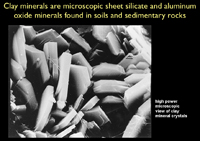
Fig. 2-92. Clay minerals (shown here in microscopic view). There are many kinds of clay minerals. |
|