Torrey Pines State Nature Reserve |
|
A1
Geology Field Guide to the Torrey Pines Park Area |
This presentation is a general overview of the geologic features and processes shaping the landscape in the "Torrey Pines park area." The named areas in discussion include the Torrey Pines State Nature Reserve and Torrey Pines State Beach, and Blacks Beach.
Figure 1 is a satellite map of the that Torrey Pines park area. Labeled features are used in the discussions below. In terms of park visitation, the beach area is the destination of most visitors (Figure 2). The beach extends along the Torrey Pines Road causeway and southward along the seacliffs to Blacks Beach and beyond to the La Jolla area. It is important to mention that visitors to the seacliff area is potentially hazardous because of rock falls and is particularly dangerous during high tides with storm wave activity (Figure 3).
The upland part of the park is popular for hiking, scenic views, and wildlife observation. The park exists because of the dedicated efforts of many conservation-minded people who worked hard to protect the endangered Torrey Pines and associated coastal shrub plant community (Figure 4). The beach, cliffs and hillsides are host to abundant wildlife: birds, reptiles, mammals, and insects adapted to the seasonably dry summers and cool, moist winters. Yes, be aware or rattlesnakes (Figure 5). They are generally docile and will try to avoid you. Visit the Torrey Pines Association website for park information, rules, trail maps, history, and details of about the park area wildlife and ecology. |
Click on images for a larger view. |
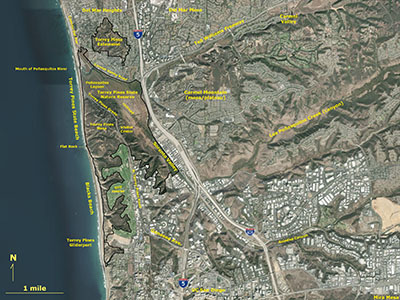
Fig. 1. Satellite features map of the Torrey Pines park area. |
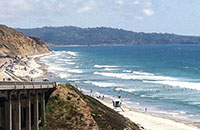
Fig. 2. View looking south along Torrey Pines State Beach with Mt. Soledad in La Jolla in distance. Wave action highlights the shallow seabed along this popular beach. |
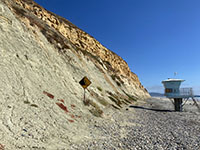
Fig. 3. This view shows the north end of the seacliff near Lifeguard Station 1 with a cliff rock-fall hazard warning sign posted. |
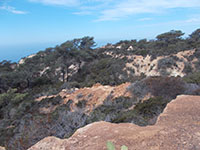
Fig. 4. The endangered Torrey Pines are part of coastal plant community protected in the upland mesa part of the Torrey Pines State Nature Reserve. |
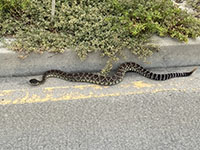
Fig. 5. Western diamondback rattlesnake along the Torrey Pines Park Road. Rattlesnakes are part of the native wildlife community in the park. |
|
A2
Geology of the Torrey Pines Area |
Figure 6 is a generalized geologic map of the region that includes the Torrey Pines park area. The colors on the map show the locations where bedrock of different ages and compositions exist at or near the surface. The location of faults, both onshore and offshore (including earthquake faults) and other topographic and bathymetric features are included on the map. Note that the Torrey Pines park area is only a small fraction of the map along the coast in the vicinity between Solana Beach and Dike Rock (show on the map). More detailed information is presented below in a general chronology starting from the oldest rocks to the modern features and processes currently shaping the landscape.
Much of the discussion below focuses on stratigraphy. Stratigraphy is the branch of geology concerned with the study of rock layers (strata) and layering (stratification). All of the rock layers in the Torrey Pines park area are sedimentary in origin, made up of fragments of older rocks—weathered, eroded, and deposited as gravel, sand, silt, and clays. The sedimentary layers exposed in the seacliffs and throughout the reserve are divided into named rock formations. To be designated as a rock formation they must be different enough from each other to be visually told apart and are big enough to be differentiated on a geologic map. |
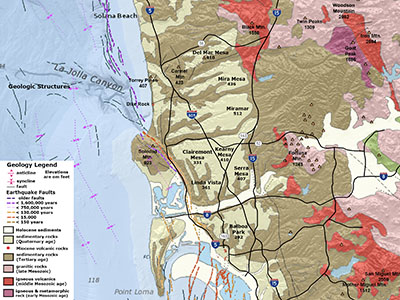
Fig. 6. Generalized geologic map of the Torrey Pines region. |
A3
Rock Formations Exposed In The Torrey Pines Area |
Figure 7 is a generalized summary of the rock formations (stratigraphy) exposed in the Torrey Pines park area. Starting with the oldest layers exposed, these included:
- Delmar Formation
- Torrey Sandstone
- Ardath Shale
- Scripps Formation
- Lindavista Formation
- Bay Point Formation
These are the names of rock formation that have been formally accepted by the U.S. Geological Survey and incorporated into the Lexicon of Geology.
The ages of the rock formations have been determined from the fossils found in each and to other locations where the age of the rocks have been determined by other means.
The Delmar Formation, Torrey Sandstone, Ardath Formation and Scripps Formation are all middle Eocene age (around 49 to 45 million years old). These rock formations are part of a much larger package of Eocene age rock formations in the coastal San Diego region collectively designated as the La Jolla Group. Figure 8 illustrates the general depositional environments where the sediments of these rock formations accumulated.
In contrast, the Lindavista Formation and Bay Point Formation are very young (geologically speaking—having been deposited under very different circumstances during periods of changing sea levels throughout the ice ages of the Pleistocene Epoch). The Lindavista Formation is middle Pleistocene (roughly 0.7 to 1.0 million years old), and the Bay Point Formation is upper Pleistocene (about 120,000 to 700,000 years old). These formations are associated with ancient marine terrace deposits that have been described in different locations in the San Diego Bay region, of which at least six are partly preserved in the park. |
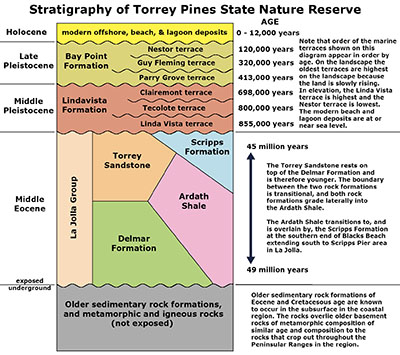
Fig. 7. Stratigraphy of the Torrey Pines Pines State Nature Reserve and park area (layers listed oldest to youngest—bottom to top). |
A4
Sedimentary Processes In Torrey Pines Area—Then And Now
Processes shaping the coastline today are basically the same as they happened in the geologic past. However, the geometry of the West Coast during Middle Eocene time (45-49 million years ago) was very different than today. What is now San Diego County during the Eocene was part of a coastal plain west of the eroding remnants of volcanic highlands of the north-central Mexico region—the Gulf of California and the San Andreas Fault system had not formed yet. Rivers draining from the Mexican uplands drained directly in the Pacific Ocean with a principal stream (the ancient Ballena River) that dumped sediments into the ocean in what is today the coastal San Diego region (Figure 8). The sedimentary features we see in the La Jolla Group (which includes the Delmar Formation, Torrey Sandstone, Ardath Shale and Scripps Formation) were formed in shallow nearshore to offshore paralic sedimentation including alluvial, lagoonal, deltaic, littoral, and shallow neritic sedimentary environments. Paralic sedimentation (from the Greek paralia, meaning seacoast) pertain to the inter-tongued marine shelf, transitional, and coastal environments and the sediments they capture.
Figure 9 also illustrates coastal sedimentary environments and features. These sedimentary features illustrate the kind of features that may have existed in the Torrey Pines park area before modern construction began in the early 20th century. The modern depositional environments have been heavily modified by the constructions of the causeways and bridges for the railroad and the Old Coast Highway (Torrey Pines Road). The flow of modern tidal channels of the lagoon is now limited to the location of the bridges. In addition, the natural supply of sandy sediment to the coastline beaches has been restricted. Seacliff erosion prevention efforts along the coast and the construction of dams on rivers and streams have reduced the sand supply that would otherwise supply sand to beaches. In an unrestricted natural environment the location of inlets and channels cutting through coastal bay-mouth bars would be constantly shifting. Now expensive dredging operations are needed to maintain channel flow and sand supply to the beach.
|
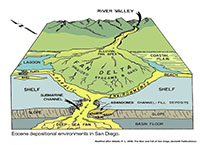
Fig. 8. Eocene depositional environments in San Diego
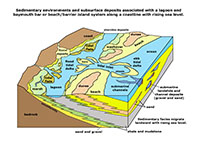
Fig. 9. Paralic sedimentary environments associated with beaches, barriers, bay mouth bars, and lagoons, and offshore |
A5
Using Sedimentary Facies To Describe Rock Formations
The discussions below describes sedimentary rock formations in the Torrey Pines park area. In geology, the term rock formation is used to describe a body of rock having a consistent set of physical characteristics (lithology) that distinguish it from adjacent bodies of rock. Named sedimentary rock formations occupy a particular position in sequence of layers of rock distributed in a geographical region. Rock formations can be exposed and occur below the surface. Giving a package of sedimentary rock layers a formal formation name makes it easy to communicate some information. However, the term sedimentary facies provides a better description of the origin of how the sedimentary material was originally formed.
Sedimentary facies are bodies of sediments (or sedimentary rocks) that are recognizably distinct from adjacent sediments (or rocks) that resulted from sediments accumulating in different depositional environments. Sedimentary facies are masses of rock that can be recognized by its composition, structures or fossil content, and can be mapped on the basis of those characteristics. Examples include beach facies, lagoonal facies, submarine channel facies, dune facies, etc. Figures 8 and 9 illustrate sedimentary facies, showing sedimentary environments actively forming on the surface and their relationship to sedimentary facies where their associated sediments are preserved below the surface. |
A6
General Geology of the Torrey Pines State Nature Reserve
The landscape and geologic features in the reserve are best examined in the context of the greater geologic history story of the San Diego Region extending back hundreds of millions of years. What is visible in the reserve area is only a small portion of the larger story as described in Plate Tectonics and Regional Geology of San Diego County. The Eocene-age rock formation form the bedrock foundation in the reserve area, whereas the younger Pleistocene age formations are essentially surface-coating deposits preserved on the eroded surface of older landscapes.
Looking south from Carmel Valley Road, the upland part of the Torrey Pines State Nature Reserve has the appearance of a mesa—an isolated flat-topped hill with steep sides, found in landscapes with horizontal strata (Figure 10). The mesa is actually part of a larger erosionally dissected plateau that includes the broader upland areas extending east from the coastline to the base of the mountains.
Figure 11 is a slightly revised version of a classic "gull's view" geologic map illustration showing the geologic features (rock formations and faults) of the Torrey Pines mesa (modified after Hubbs and others, 1991). Figures 12 and 13 illustrate generalized east-to-west cross-sections of the Torrey Pines mesa.
|
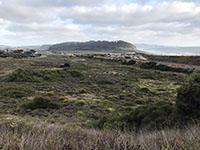
Fig. 10. View looking south towards Torrey Pines mesa |
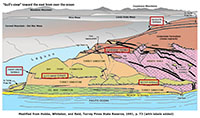
Fig. 11. A "gull's view" of the geology of Torrey Pine State Nature Reserve (TPSNR) |
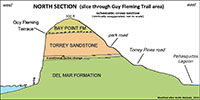
Fig. 12. East-to-west cross section at the north end of the mesa in the TPSNP in vicinity of the visitor center |
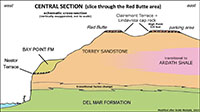
Fig. 13. East-to-west cross section at the central section of the TPSNR in the vicinity of the Guy Fleming Trail |
|
A7
Rock Formations Exposed Along the Sea Cliffs of Torrey Pines and Blacks Beaches |
The series of images below show an interpretation of how the Eocene-age rock formations (Delmar, Torrey, Ardath, and Scripps formations) express themselves in the sea cliffs in the Torrey Pines and Blacks Beach area between the Peñasquitos Lagoon (north) and Scripps Pier (south). The rock formations have unique characteristics that express themselves in appearance—lithology, weathering style (cliff vs. slope forming), and superposition. In some locations, the boundary between formations is transitional, either laterally or vertically. These subjective formation boundaries should be redefined by facies. |
Figures 14 to 21. Interpretation of the outcrop belts of Eocene-age rock formations and features in the sea cliffs along Torrey Pines Beach and Blacks Beach between Peñasquitos Lagoon and Scripps Pier in La Jolla. Peñasquitos Lagoon is in the upper left, Scripps Pier is on the lower right. Base images were modified from earth.google.com. |
Fig. 14.
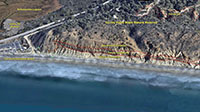 |
Fig. 15.
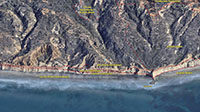 |
Fig. 16.
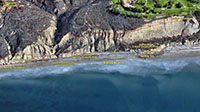 |
Fig. 17.
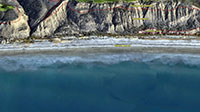 |
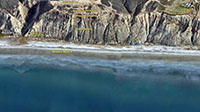
Fig. 18. |
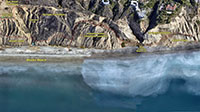
Fig. 19. |
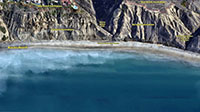
Fig. 20. |
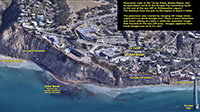
Fig. 21. |
|
A7
Stratigraphy of the Torrey Pines State Nature Preserve
The discussion below describes the general character of the different rock formation exposed in the Torrey Pines park area. Figure 22 show the exceptional exposures of rock formation in the sea cliffs above the beach. Note that there are many other named rock formations in the San Diego area, including many that exist in the subsurface below the modern landscape features. Those exposed in the Torrey Pines State Nature Reserve and along Torrey Pines State Beach and Blacks Beach are discussed below.
The first part of the discussion focuses on the Eocene age rock formations: the Delmar Formation, Torrey Sandstone, Ardath Shale, and Scripps Formation. The next part of the discussion focuses on the much younger Pleistocene age marine terrace deposits (Lindavista and Bay Point Formations) that locally blanket (unconformably overlie) the older rock formations. |
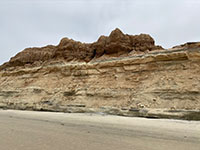
Fig. 22. View of rock formations exposed in the sea cliff at Torrey Pines. |
A9
Delmar Formation
The Delmar Formation is exposed only at the base of the sea cliffs from just south of the main reserve entrance and parking area to just south of Flat Rock, —a small sea stack adjacent to a small headland in the sea cliff that defines the boundary between Torrey Pines State Beach (to the north) and Blacks Beach (to the south). The Delmar Formation is exposed in the lower seacliffs from Torrey Pines northward to parts of Del Mar, Solana Beach, Encinitas, and Carlsbad. Elsewhere it is not exposed (below the surface), it transitions to another named rock unit, or it is missing (eroded away or never deposited).
When walking south along Torrey Pines Beach starting from near the Park Entrance Station and Lifeguard Station #1 goes through some physical changes. Near the Lifeguard Station, the The Delmar Formation consists mostly of a greenish or yellow mudstone and siltstone (like sandstone but with smaller grains) (see Figures 23, 24, and 25 below). As you head south along the beach, some beds transition into more consist massive and thicker beds of sandstone. Midway down the beach the boundary between the green Delmar Formation and the overlying massive, buff-colored Torrey Sandstone is quite distinct (Figure 26). Farther south along the beach, the boundary between the underlying Delmar Formation and overlying Torrey Sandstone becomes more "transitional" with layers of sandstone and mudrocks beginning to inter-layered with one another (Figures 27 and 28).
Starting about midway south along the beach layers of sandy marl fossil beds appear in the lower seacliff of the Delmar Formation. The fossil beds display an abundance of oyster shells (some hole, most as fragments) with some other scarce pelecypods and gastropods (Figures 29, 30, and 32). Some layers lack fossils but preserve an abundance of bioturbation features, including interconnected burrows typical of small crustaceans (but their body fossils are not preserved; Figure 32). The oyster-bearing beds are mostly tightly cemented and protrude as more resistant layers along the base of the seacliff. By comparing the life habit of oysters in modern coastal settings, it can be assumed that sediments of the Delmar Formation were deposited in a lagoonal setting.
Fragments of fossil wood, casts of logs, or thin local layers of coal also occur in some locations, particularly in the transition zone—an example in the seacliff near Flat Rock is shown in Figure 33. In this case, the logs have decayed away, but a concretion layer had formed around the logs.
There is also an abundance of sedimentary features that display the migration and deposition of sediments in shallow-water, coastal settings. Thinly laminated beds that lack bioturbation reflect quiet or stagnant water conditions in some beds typical of a lagoonal setting (Figure 34). Some beds preserve cross-bedding that preserve information about the direction the water was flowing as it transported sandy sediments (Figures 35 and 36).
Flat Rock is a small sea stack of resistant sandstone beds of the lower Delmar Formation. Flat Rock is located next to a small headland in the seacliff that divides During high tide or stormy conditions this area can be quiet dangerous (Figure 37). A small trail runs low around the headland in the seacliff that provides passage between the two beaches (Torrey Pines State Beach to the north, and Blacks Beach to the south). In the seacliff at Flat Rock it is easy to see the side of an ancient submarine channel, possibly a tidal delta channel, that has filled in with sediments (Figure 38). Figure 39 shows detail of the ancient soft-sediment erosion features in the channel fill deposits including both soft pebble (oddly shaped clasts) and flat pebble (flat chip-like clasts) preserved in a matrix of coarse sandstone. Flat pebble conglomerates are commonly associated with material eroded from desiccated mud flats that have been ripped up and transported by storm currents.
South of Flat Rock, the lower fossil-bearing beds of the Delmar Formation gradually disappear below beach level and the upper transitional sandstone beds merge laterally into massive sandstone beds characteristic of the Torrey Sandstone (Figures 40 and 41).
Figure 42 is a view of the Delmar Formation in its type area located at the Del Mar Dog Beach, North Point Park in Del Mar, CA.
|
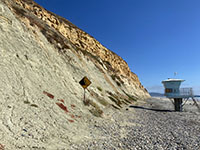
Fig. 23. Greenish mudrock (sandy-shale beds) exposed near the end of the seacliff at Lifeguard Station #1 on Torrey Pines State Beach.
|
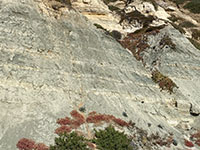
Fig. 25. Cyclic bedding of mudrocks of the Delmar Formation exposed in the seacliff reflect possible cycles in the rise and fall of sea level in Eocene time. |
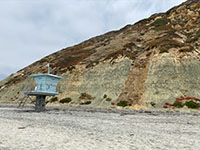
Fig. 26. Greenish shaly sand beds (mudrocks) of the Delmar Formation form the seacliffs near near Lifeguard Station 1 on Torrey Pines State Beach.
|
Fig. 26. Delmar Formation (green) overlain by Torrey Sandstone (brown) form the seacliff for several hundred yards south of the Lifeguard Station #1. |
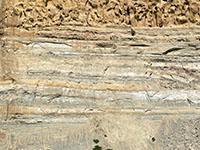
Fig. 27. As you walk south along the seacliff, the mudrocks gradually transition to more sand-rich layers in the zone below the more massive Torrey Sandstone. |
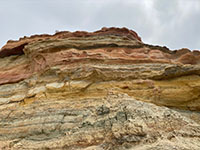
Fig. 28. Brightly-colored beds in the transition zone between the Delmar Formation and the Torrey Sandstone near where the Beach Trail descends to the beach.
|
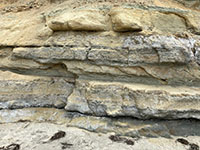
Fig. 29. Shell beds containing mostly oysters, some gastropods, and other uncommon pelecypods occur in the lower beds of the Delmar Formation. |
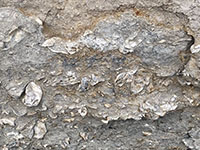
Fig. 30. Randomly arranged whole and fragmented oyster shells in a marly sand bed suggest rapid deposition, possibly storm or tsunami related. |
Fig. 31. Several fossiliferous beds of marly sandstone occur in the Delmar Formation, oyster shells being the most common fossils. |
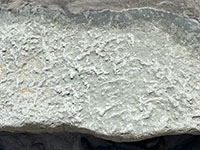
Fig. 32. Some beds display bioturbation (burrow), probably by small crustaceans whose remains are not preserved. |
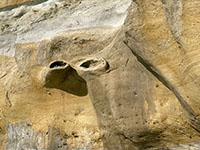
Fig. 33. Fossil log casts protrude from the sandstone seacliff in the Delmar/Torrey transition zone near Flat Rock.
|
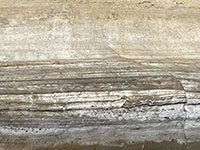
Fig. 34. Finely laminated beds, lacking bioturbation, reflect quieter, restricted water setting for these beds in the Delmar Formation on Blacks Beach.
|
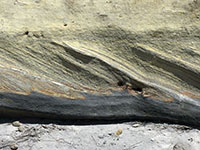
Fig. 35. Cross-bedded sandstone layers are visible in many locations in the Delmar Formation along the lower seacliffs.
|
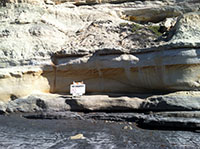
Fig. 36. This image shows a distinctly colorful layer of cross beds highlighted by limonite cement preserved in Delmar Formation.
|
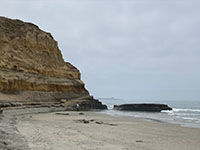
Fig. 37. Delmar Formation in the lower sea cliff near Flat Rock. The Flat Rock sandstone beds are tightly cemented and more resistant to erosion. |
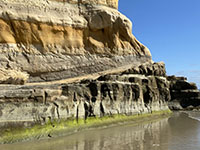
Fig. 38. An ancient channel filled with oyster beds and sandstone cuts into older beds of the upper Delmar Formation near Flat Rock.
|
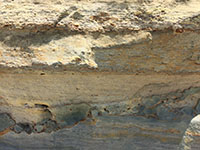
Fig. 39. Soft-pebble and flat pebble conglomerate and seabed erosion features in the Delmar Formation near Flat Rock. |
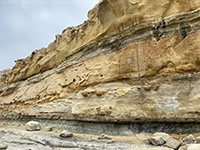
Fig. 40. Round concretions in the transition zone between the Delmar and Torrey Sandstone Formations.
|
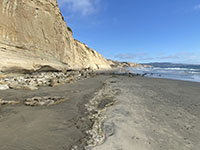
Fig. 41. Layers of the Delmar Formation gradually dip and disappear below the surface of Blacks Beach south of Flat Rock. |
Fig. 42. Delmar Formation transitions to Torrey Sandstone at Del Mar Dog Beach, North Bluff. Bay Point Formation tops out the bluff. |
|
A10
Torrey Sandstone
The Torrey Sandstone is exposed as massive white-to-buff gray cliffs throughout the Torrey Pines area. It is well exposed in road cuts along the Torrey Pines Grade (it's designated type section for comparison with other locations (Figures 43). It crops out along the upland canyons such as along the Guy Fleming Trail where the massive sandstone beds are almost 100 feet thick (Figure 44). It is also well exposed along the upper seacliffs above the beaches—both Torrey Pines State Beach and the northern end of Blacks Beach (Figures 45).
The Torrey Sandstone consists of cemented sand composed dominantly of quartz, lesser feldspar, and traces of iron-rich minerals (biotite, magnetite, and other mafic minerals). The sand is mostly fine- to medium-grained, well-rounded, wave-washed sand (beach and bar sand deposits) with some coarser, more angular-fragmented river sand materials mixed in in some areas. It is the weathering of the iron-rich minerals that produces the rust-like colors to weathered surfaces. In its various forms, the mineral limonite (FeO(OH)·nH2O,) is what gives the weathered rock and soil derived from it is tan, buff, reddish-brown, and orange colors. Limonite along with silica (SiO2), calcite (CaCO3), and clay minerals deposited by groundwater that cemented the sands together.
The massive bodies of sand in the Torrey Sandstone are generally featureless (massive) or locally preserve cross-bedding created by flowing currents. Pervasive bioturbation by benthic organisms have churned some sedimentary beds, destroying all bedding structures, but their fossil remains are not present or not preserved. The massive sandstone bodies represent a mix of sub-aerial depositional environments of offshore bars, sediment-filled channels, tidal delta deposits, but aerial-exposed deposits (beach, barrier, dune, and baymouth bar) are generally not preserved or represented locally (see Figures 8 and 9).
The Torrey Sandstone exposed in the seacliffs display an unusual style of weathering and erosion called tafoni (Figure 46). Tafoni forms on surfaces of sandstone (and other porous rocks) where slightly salty rainwater seeps in the rock. As it seeps through the rock it dissolves some of the mineral content of the sand and cement holding the sand grains together. Then, as the weather dries out, some of the water is drawn back out to the surface where it evaporates, leaving the dissolved mineral content behind. This results in case-hardening of the surface, whereas the sandstone below the surface looses its tightly consolidated character. As fracture or gap in the case-hardened surface appears, the hole creates a space for the softer sand below the surface to dry, crumble, and be blown out by the wind. This enlarges the holes over time to create the irregular-shaped or honeycomb-shape surfaces associated with tafoni-style weathering. The holes also trap and hold moisture condensate (dew)from the air, which helps to weather the sand inside the hole. Basically same process is responsible for the formation of small wind caves found along the canyons in the Torrey Sandstone throughout the reserve area. If you sit inside one of these holes, you may observe the slight rain of sand grains drying out and falling from the roof of the small caves.
The Torrey Sandstone have an abundance of concretions, but the ones in the Torrey Sandstone really stand out. The are rounded, ball- or sphere-shaped nodules, up to about a meter in diameter (Figures 47 and 48). The concretions began to form not long after the sediments were deposited and were cause by the deposition of calcite and iron-oxide cements precipitated by groundwater. Concretions tend to nucleate on something that causes a local change in the groundwater chemistry. Lab studies of how concretions form show that decaying protein-rich matter releases ammonia which can cause calcite or limonite to start to precipitate in the gaps between sand grains. Once started, the crystallization process spread into the surrounding porous sand creating the spherical shape. Some concretions contain shells which are typically not crushed compared to shells in the surrounding rock outside of the concretions. This shows that the concretions can form earlier than the burial and compaction of the surrounding sediment. Over time, the concretions can build up a rind-like appearance, similar to growth rings on a tree. Concretion rinds can also form round sticks and logs (such as illustrated in Figure 33).
The Torrey Sandstone also displays evidence of ancient landslide deposits and contorted bedding created where material form the sides of a submarine channel collapsed and filled in the base of a channel (Figures 49 and 50). The Torrey Sandstone actually consists of sedimentary material, mostly sand, deposited in a range of coastal and offshore sedimentary environments including beach or barrier dunes, shallow marine shoals or sand bars, tidal delta, and shallow shelf channel fill deposit. These grade laterally into finer-grained sedimentary deposits of the Delmar Formation and the Ardath Shale (discussed below).
|
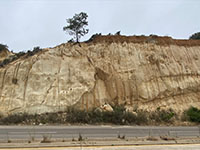
Fig. 43. The type section for the Torrey Sansone in the road cut along Torrey Pines Grade (Torrey Pines Road, or Old Coast Highway).
|
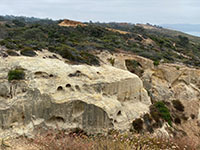
Fig. 44. Massive outcrops of Torrey Sandstone occur along some of the park's canyons. This one is on the north side of the Perry Grove Trail.
|
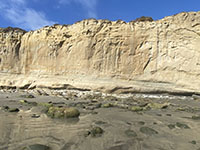
Fig. 45. The Delmar Formation transitions upward into the massive sandstone bodies of the Torrey Sandstone such as exposed here at the north end of Blacks Beach. |
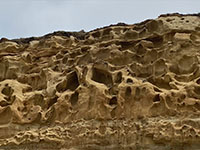
Fig. 46. Tafoni-style weathering features dominate the exposures of the Torrey Sandstone in the seacliffs along Torrey Pines State Beach.
|
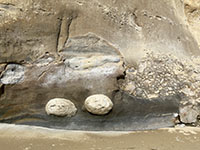
Fig. 47. Large sandstone concretions and soft-pebble landslide deposits in the lower Torrey Sandstone exposed in an ancient submarine channel deposit along Blacks Beach.
|
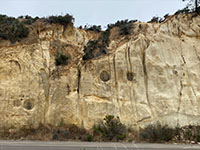
Fig. 49. Large brown concretions in the massive exposure of Torrey Sandstone exposed in the road cut along Torrey Pines Road (road grade).
|
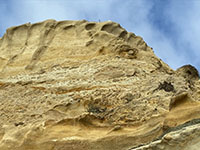
Fig. 49. Submarine landslide deposits filling an ancient seabed channel in the lower Torrey Sandstone along Blacks Beach. Here the rocks are very similar to the Scripps Formation.
|
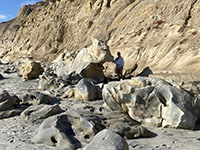
Fig. 50. Large irregular-shaped (contorted) blocks of sandstone formed from soft-sediment deformation in a submarine channel are eroding from the Torrey Sandstone-Ardath Shale on Black Beach. |
|
A11
Ardath Shale
The Ardath Shale crops out in the seacliffs in the southern part of the Torrey Pines State Nature Reserve and along Blacks Beach. It consists dominantly of poorly consolidated fine sandy, silty, to shaly mudrocks (Figure 51). Not all of the Ardath Shale is composed of shale (Figure 52). It forms the massive barren slopes above Blacks Beach (Figures 53, 54, and 55). It is transitional laterally with, and overlies, some of the massive sandstones of the Torrey Sandstone. The Ardath Shale also has large channel sand bodies with in it that locally preserve massive underwater landslide deposits (Figures 56 and 57). Further south along Blacks Beach the Ardath Shale transitions to, and underlies, a more massive sandstone and conglomerate-bearing unit named the Scripps Formation.
Abbot (1999) describes the entire section of Ardath Shale outcrops exposed in the from Torrey Pines southward to Scripps Pier essentially a large submarine channel complex of middle Eocene age that has been uplifted to its current position. The submarine channel fill and landslide deposits contain gravel clasts that correlate to the Poway Group gravels, an Eocene river and alluvial fan complex preserved in patches in the upland regions between San Vicente Reservoirs and Ramona. These gravels were deposited by the ancient Ballena River—a river system that drained from the volcanic uplands of the Sonora Region of Mexico and drained to the ancient coastline of San Diego (see Figure 8). This ancient river system existed before plate-tectonic forces rifted open the Gulf of California and formed the modern San Andreas Fault system. In Eocene time, the highlands that are now the mountainous regions of eastern San Diego County were much lower, gently rolling plain that was much closer to sea level. As the Gulf of California formed, the Ballena River eventually vanished and the landscape has slowly risen to its current heights and broken by the numerous faults that now cut through the San Diego region.
The Ardath Shale consists a mix of shale, silty shale, with some beds of sandstone and conglomerate that are similar in physical appearance to some beds in the other Eocene-age rock formations. However, the Ardath Shale is dominated by steep-slope-forming shale (not the nearly vertical cliffs of the sandstone and conglomerate beds). The soft shale beds erode easier forming alcoves beneath waterfalls (Figure 57) and is susceptible of forming slumps (Figures 58 and 59).
The Ardath Shale probably was deposited in deeper water offshore where waves and current energy allowed the finer sediment fractions to accumulate. The Ardath shale has intermittent wedge-shape sand bodies with some conglomerate beds that represent deposition in submarine channels that filled with submarine landslide deposits and coarser sandy material transported by turbidity currents draining off the continental shelf from the ancient Ballena River delta. The Ardath Shale transitions to the Scripps Formation towards the southern end of Blacks Beach (Figure 60).
|
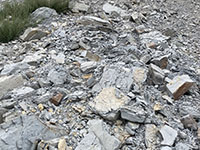
Fig. 51. Shale exposed in a fresh landslide that tumbled from the Ardath Shale along Blacks Beach.
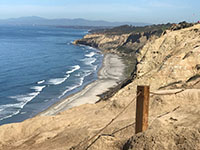
Fig. 52. View looking north along the seacliff composed of Ardath Shale at Blacks Beach at the south end of Torrey Pines State Nature Reserve as seen from the Torrey Pines Gliderport area. |
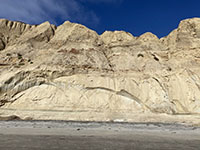
Fig. 53. Massive beds of Torrey Sandstone are overlain by softer mudrocks of the overlying Ardath Shale exposed in the sea cliff at the north end of Blacks Beach. |
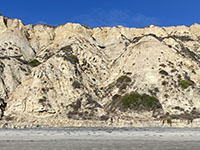
Fig. 54. Massive eroding slopes and seacliffs composed of the Ardath Shale are exposed along Blacks Beach at the south end of Torrey Pines State Nature Reserve. |
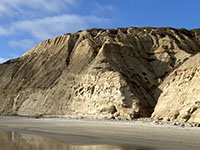
Fig. 55. Ardath Shale exposed in the seacliff and ravine on the south side of Broken Hill Overlook along Blacks Beach.
Next image shows detail at the mouth of the ravine. |
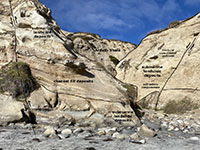
Fig. 56. Ancient submarine landslide and channel fill deposits in the Ardath Shale at the mouth of a ravine south of Broken Hill Overlook on Blacks Beach. |
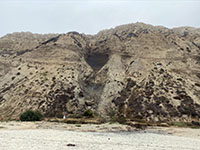
Fig. 57. Alcoves in the sea cliff on Blacks Beach form by the selective erosion of the softer shale beds in the Ardath Shale. Harder sandstone beds form ledges. |
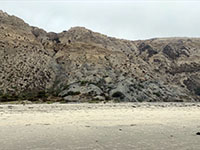
Fig. 58. Gray shale beds in the Ardath Shale on Blacks Beach are susceptible to landsliding. This older slumps at the base of the sea cliff area is just north of Blacks Beach Park |
Fig. 59. View looking north at the hummocky slopes of old slump material that accumulated on the slope below sea cliffs of Ardath Shale on Blacks Beach. |
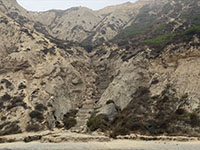
Fig. 60. The Ardath Shale basically intertongues with—or transitions to—the Scripps Formation south of the Gliderport Trail on Blacks Beach. |
|
A12
Scripps Formation
As you walk north on the beach from Scripps Pier in La Jolla, a seacliff rises from near sea level to nearly 100 meters (330 feet). The entire rock sequence exposed in the seacliff has been assigned the named Scripps Formation and its type area was designated in a canyon near the south end of Blacks Beach about 1 kilometer north of Scripps Pier (Figure 61). The Scripps Formation extend northward as a massive wedge of sediments for about 1.5 miles consisting mostly as massive bed of conglomerate and sandstone (Figure 62). North of the type area hat gradually transitions into and overlies the Ardath Shale midway to the northern end of Blacks Beach. Canyons cutting bisecting the sea cliff produce the rugged scenery in the vicinity of Torrey Pines City Beach (Figures 63, 64, and 65).
The sediments of the Scripps Formation have characteristics that support an interpretation that they were deposited in an offshore setting associated with a river delta fed coarse-grained that poured sediments onto the continental shelf into submarine channels or canyons that extended into a deeper water settings father offshore along the continental shelf margin of an Eocene fore-arc basin (see Figure 8). Some of the sediments were deposited in turbulent, high-energy settings, resulting in beds of conglomerate (Figure 66), ripped-up materials and beds of contorted sediment associated with submarine landslide deposits (Figures 67 and 68). Massive pod-shaped beds of sandstone appear to fill in channels cut into older finer-grained sediments originally deposited in quieter-water settings. The Ardath Shale represents those quieter water settings farther away (north) along the continental away from the delta complex of the ancient Ballena River system.
|
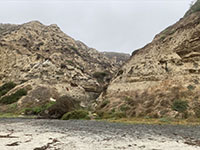
Fig. 61. The Scripps Formation type area is located in a canyon along Blacks Beach about 1 kilometer north of Scripps Pier. |
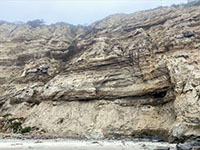
Fig. 62. Massive beds of conglomerate and sandstone of the Scripps Formation just north of Dike Rock at the southern end of Blacks Beach. |
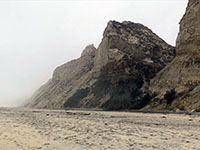
Fig. 63. View looking north on Blacks Beach to a large butte of sandstone and conglomerate at the end of the La Jolla Trail Overlook. |
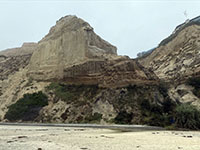
Fig. 64. Massive outcrops of sandstone and conglomerate of an ancient submarine channel deposit near the Saigon Trail canyon on Blacks Beach. |
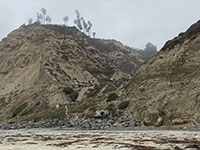
Fig. 65. Scripps Formation crops out in the sea cliff and canyon along the Torrey Pines City Beach Trail to Blacks Beach. |
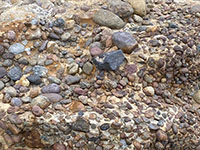
Fig.66. Conglomerate in the Scripps Formation in an outcrop near Dike Rock is composed mostly of gravel of volcanic origin. |
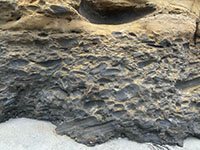
Fig. 67. A flat-pebble conglomerate composed of rip-up clasts deposited in a submarine landslide deposit in the Scripps Formation. |
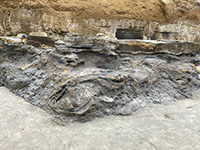
Fig. 68. Contorted layers in a shale bed beneath a hard sandstone layer of a wave-cut bench. This exposure is at the north end of Scripps Beach. |
Fig. 69. A concretion showing spherical growth rings. Concretion similar to this one occur in sandstone beds of all the Eocene rock formations. |
|
A13
A Gap In the Rock Record At Torrey Pines—Eocene to Pleistocene
It should be noted that depositional processes continued in the San Diego region after the deposition of the Eocene-age sediments representing the the Delmar, Torrey, and Ardith formations, but they are not preserved in the park area. There is significant gap in time represented by the unconformities between the rock formations of Eocene age and those of Pleistocene age—a gap of about 44 million years—representing a long period of non-deposition or erosion of materials that may have once covered the region. Sediments and volcanic ash beds of Early Oligocene age (30-28 million years ago) are preserved as the Otay Formation in the southeastern part of San Diego County. What is inferred from evidence here and elsewhere around the world was that there was a significant drop in sea level in late Oligocene time (Brown, 1996). This means that the land along the coastline and shallow marine settings would have been exposed to sub-aerial erosion.
Perhaps more important were the big changes in the plate-tectonic setting of the region began in Miocene time. The western coast of the central and northern Mexico began to bulge and rift apart. This slowly creating the spreading center and transform faulting that would eventually flood to create the Gulf of California and the San Andreas Fault system in southern California (Figure 70). This tectonic activity is what created the Peninsular Ranges of Southern California and Baja. Before this happened, the region that is now the mountainous areas of San Diego County was a low, eroded coastal plain. The surface of this ancient plain s is partly preserved in the high country plateaus of eastern San Diego County).
Some sediments preserved as the San Onofre Breccia on Camp Pendleton (northern San Diego County) that it contains material that was eroded from ancient ocean crust that is now exposed on Catalina Island and other parts of the Channel Islands. These sediments are about 17-14 million years old—Early to Middle Miocene age (Tan and Kennedy, 1996). These sediments indicate that Catalina Island and the other islands and seamounts off the coast of Southern California (large fault-bounded blocks of crust) were at one time attached to the San Diego coastline. Geologists named this offshore region the Borderlands (Figure 71).
Another clue about what was happening in Miocene time was the intrusion and eruption of volcanoes along the San Diego coastal region. Three locations where remnants of these volcanoes are preserved include the Morro Hills volcanic field (near Bosnall), The Calavera Hills volcano (in Carlsbad), and nearby, Dike Rock exposed on the beach between Blacks Beach and Scripps Pier see Figure 72; also the location of Dike Rock on Figure 21). These volcanic areas show that molten material deep within the crust found passage to the surface along the regional developing fault system. These small volcanic areas and their inactive faults possibly represent “aborted rift zones” that did not continue to split and move apart and away like the ones that created the basins, seamounts and islands that exist offshore (shown in Figure 71). Basically, a coastal plain that existed along the San Diego coastline in Miocene was torn apart. The numerous earthquake faults throughout the San Diego region are a continuation of that plate-tectonic activity.
The fault systems in Southern in California remain active, but some dynamics have changed. By Pliocene time (about 3 million years ago), two large, shallow embayments developed along parts of the coastal region in San Diego. One was south of La Jolla and extended inland along the coast, and another formed up north in the Camp Pendleton region. Shallow marine and coastal alluvial deposits are preserved in the region from those times, but no sediments from that time period are preserved in the Torrey Pines area. |
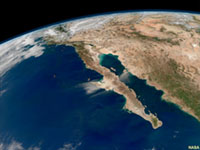
Fig. 70. Satellite view of Baja California and the southern end of the San Andreas Fault.
Fig. 71. Map showing offshore bathymetry of the Southern California Borderlands that consists of large fault-bounded blocks associated with with seamounts, basins, and islands.
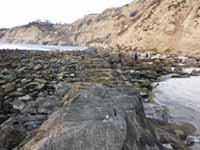
Fig. 72. Dike Rock is a dark volcanic rock exposed along the southern end of Blacks Beach. The sea cliffs in the background are Scripps Fm.
|
Note that Dike Rock is one of the largest accessible tide pool areas along the coast north of La Jolla. Be caution when hiking through this area because the rocks may be unstable. For a good summary tide pool sea life, see Life in the Rocky Intertidal Zone (Cabrillo National Monument brochure). |
A14
Ice Ages of the Pleistocene: Impact on the San Diego Area (Torrey Pines) |
The Ice Ages of the Pleistocene Epoch began about 2.4 million years ago, and the last ice age ended about 11,500 years ago (although they are still occurring in Greenland and Antarctica, at least for now). In that time period there were at least 16 glaciations (possibly as many as 20 have been suggested). A glaciation is the process or result of large areas of land being covered by extensive polar ice sheets. At the peak of the glaciations, continental ice sheets covered much of northern North America, northern Europe, Siberia, and ice caps covered many mountain ranges around the world (Figure 73). Some of these glaciation periods lasted longer and were more extensive than others.
Likewise, as glaciation cycles ended, the melt waters flowed back into the ocean. Like the glaciation periods, some of the interglacial periods lasted longer and were more intense than others (Figure 74). Whereas the San Diego coastline has become relatively geologically stable (somewhat), sea level began to fall and rise with the cycles in the formation and melting of the polar ice caps. Estimates vary, but when the massive continental glaciers formed (on land) sea level fell as much as 400 feet lower, and when they melted, sea level rose as much as 80 feet higher relative to
current sea level.
These cycles in sea level changes are erosionally superimposed onto tectonically rising blocks of land, resulting in the formation of marine terraces.
Marine Terraces
Figure 75 shows an undeveloped coastline on San Clemente Island that preserves a series of elevated marine terraces and associated sea cliffs along rising coastlines. Figure 76 shows how each marine terrace is linked to a peak in high-standing sea levels during the interglacial periods between glaciations. Figure 77 illustrates how wave-cut platform and seacliffs form when sea level is slowly rising, about the pace the land is rising—tectonic forces (including earthquakes) over time cause sections of the coastline to rise. When the next glaciation cycle begins, sea level falls relative to the rising land surface, so the shoreline rapidly retreats seaward while the land continues to rise. The old wave-cut platform becomes a new marine terrace. As a result, each fall and rise in sea level creates an new "step" (marine terrace and seacliff) along a rising shoreline.
Figure 78 shows features comparing the modern wave-cut platform and sea cliff to a marine terrace. Old wave-cut abrasion platforms where the ocean cut steps can be recognized by layers of wave-polished and rounded cobbles that accumulated by density of the shallow seabed. Cobbles tossed by waves was part of the action that initially carved the wave-cut platforms and adjacent sea cliffs. Layers of rounded beach cobbles are locally preserved at at the base of the younger beach sand, dune sand, and alluvial sediments that buried the steps (wave-cut platforms). The older "steps" (wave-cut platforms with seacliffs) are not obvious in some places because they have been mixed and covered with slope wash and stream sediments (sand, mud, and bedrock fragments; alluvium and colluvium). Stable areas have a modern soil profile with plant cover. They cut by canyons, eroded into badlands, and in some places, locally faulted (discussed below).
Geologists have mapped locations of sixteen elevated marine terraces in Southern California region, many are named for features in the Torrey Pines area (Figure 79; after Kennedy & Tan, 2005). Terraces are determined by detailed mapping and elevation measurements of ancient terrace shoreline and wave-cut platforms. Perhaps the easiest way to see the marine terraces is to look south from Torrey Pines Beach or a park overlook towards La Jolla. The stairs-like steps are clearly visible highlighted along the skyline (Figure 80). In Torrey Pines Reserve, they found the following terraces: Linda Vista, Tecolote, Clairemont, Parry Grove, Guy Fleming, and Nestor (oldest to youngest, respectively; see the terrace locations indicated on park area map locations in Figure 11). The younger Bird Rock and St. Louis marine terraces features may be partially preserved along Peñasquitos Lagoon and possibly in small locations along Black Beach down to La Jolla. These marine terraces (and associated sedimentary deposits) have been dated to correspond to two named rock formation: the Lindavista Formation of early Late Pleistocene age (about 800,000 to 1.5 million years), and the Bay Point Formation of Late Pleistocene age (younger than 800,000 years). These two formations are discussed below.
|
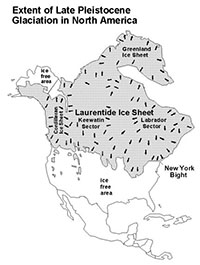
Fig. 73. Map of the extent of the continental ice sheet during the last Ice Age. |
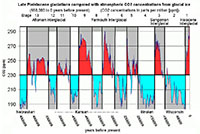
Fig. 74. Glaciation cycles (with warm interglacial periods) in the late Pleistocene Epoch inferred from carbon dioxide isotope data derived from shell material in marine sediments and ice core data. These isotope changes are linked to changes is seawater chemistry as sea level changes. |
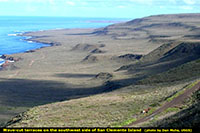
Fig 75. A series of elevated marine terraces and sea cliffs are well preserved on San Clemente Island (near San Diego). Lack of development has preserved these landscape features. A new wave-cut bench and seacliff is forming now as sea level rises. |
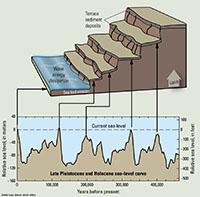
Fig. 76. Sea-level curve and origin of marine terraces. Each wave-cut platform and a sea cliff are associated with high-standing sea level of each glaciation cycle. |
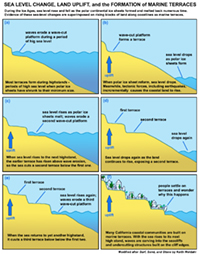
Fig. 77. How terraces form along a slowly rising coastline with changing sea level cycles. |
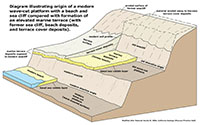
Fig. 78. Features associated with formation of a marine terrace.
Waves carve a wave-cut platform and associated seacliff. While near sea level basal gravel layers and beach deposits may be preserved. As they become elevated, material washing down from above blankets the beach deposits. |
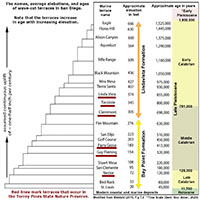
Fig. 79. Marine terraces in the San Diego region (with elevations and ages). Terraces in the Torrey Pines area are underlined in red. |
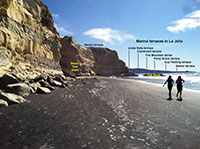
Fig. 80. The easiest way to see marine terraces is from a distance. This view is looking south near Flat Rock into the distance toward the step-like terraces in the La Jolla are on Mt. Soledad. The Nestor terrace surface is visible in the headland above Flat Rock. |
|
A15
San Diego's Mesas And An Erosionally Dissected Plateau
Figure 81 is a view looking east from a trail overlook in the Northern Extension part of the Torrey Pines Nature Reserve. The view shows the vast mesa region that extends eastward to the foothills of the Peninsular ranges in the distance. There are many communities in this region that include the word mesa in their name including Del Mar Mesa, Kearny Mesa, La Mesa, Linda Vista Mesa, Mira Mesa, North Clairemont Mesa, and Serra Mesa (to name a few). These mesas are part of a larger erosionally dissected plateau. The pale yellow-buff-colored area labeled "sedimentary rocks (Quaternary age)" on the geologic map (see Figure 6) basically shows the extent of the relatively flat upland region that has been cut by many of the principal streams draining for the mountains.
This plateau region alternated between being seafloor on a submerged continental shelf and an exposed coastal plain as sea level fluctuated up and down between about 1.5 million years ago to about 800,000 years ago. After that, the plateau region had finally rise above the reach of waves. Regional tectonic uplift has elevated the plateau region which averages a little more than about 400 feet in elevation (and a little higher to the east near the mountain front). When sea level dropped stream erosion is carving canyons that cut through the plateau. The Torrey Pines upland is a mesa within the larger plateau. |
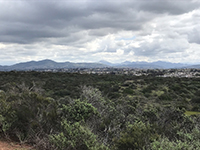
Fig. 81. View looking east at Linda Vista Mesa east of Torrey Pines. The erosionally dissected plateau (mesas) were carved into an ancient seafloor about a million years ago. |
A16
Lindavista Formation
The Lindavista Formation (original, official USGS name) or Linda Vista Formation (alternative name in literature) is the hard caprock on the plateau (mesas) region between the coast and the western base of the western foothills (shown in Figure 81). It is also the red cap rock on top of the flat upland areas in the Torrey Pines park area (Figures 82, 83, and 84). The Lindavista Formation and is associated cap-rock surfaces is one of the most significant physiographic feature in the San Diego Region, for many reasons. It is mostly the Lindavista Formation that underlies the region is shown in pale yellow on the geologic map. Note the elevations of towns (mesas) locations shown on the map. They are all in the range of about +/-400 feet. Much of the urban development in the greater San Diego area has taken place on these elevated surfaces. it is remarkable to think that about a million years ago during high-standing seas that this region was submerged as part of the continental shelf! The cycles of sea-level rise and fall and marine terrace formation was in full swing. In addition the San Diego coastline has risen about 400 feet in the last million years, or about 1/2 inch per century. Sea level is currently rising about 8-12 inches per century (depending on location).
Marine Terrace Deposits of the Lindavista Formation
Figure 85 uses the elevation data for named marine terraces in the Torrey Pines area to map the probable location of existing and potential outcrops of the various marine terrace levels. Note that this isn't a geologic map (per se) but rather a potential outcrop map because marine terrace deposits may not exist or be preserved in all these locations shown as colors on the map.
Figure 82 is a view across the valley showing the prominent cap rock layer of the Lindavista Formation on top of Torrey Pines mesa. Hubbs and others (1991) show the red cap rock of the Lindavista Formation as resting on the Clairemont Terrace at High Point (elevation 325 feet)(Figure 83). Both Red Butte and Broken Hill have similar elevations nearby in the park (Figure 84). The highest point on Torrey Pines mesa is about 410 feet in the vicinity of National University south of the park (see Figure 85). Hubs and others (1991) also show the Tecolote Terrace is also observable nearby (see Figure 6). Based on the elevation and age data elevation data shown of Figure 79 and mapped in Figure 85, this would indicate that the Linda Vista marine terrace should be in the park area.
Based on relative and absolute age dating of the marine terraces reported by Kennedy and Tan (2005), the Clairemont, Tecolote, and Linda Vista marine terraces formed between about 855,000 to 700,000 years ago (during middle Late Pleistocene Epoch (see Figure 79).
The marine terrace deposits presents somewhat of a paradox that contradicts one of the Basic Geology Principles (first reported by Charles Lyell and James Hutton in the early 19th Century), the law of superposition states that in any undisturbed sequence of rocks deposited in layers, the youngest layer is on top and the oldest on bottom. The problem with marine terraces is that oldest ones are found higher than the younger ones because of ongoing uplift. However, the marine terraces are exempt because another basic geologic principle, the law of cross-cutting relationships basically states that any fault, fold, igneous body, or other feature that cuts across another geologic feature must be younger than any rock across which it cuts. In the case of marine terraces, the uplift along the coastline is ongoing, as well as the erosion and down-cutting of features degrading the older terrace deposits over time. The highest (oldest) marine terraces show the highest degree of weathering and erosion, in some cases becoming hardly recognizable or vanishing completely.
Perhaps the best exposures of the Lindavista Formation are in the fresh road cut along the grade on Torrey Pine Road. The exposures in that cut indicate that at least two terrace-forming cycles are preserve in close succession based on the repeated layers of basal conglomerates and overlying sandstone beds (Figure 86). In the case of Figure 87, it appears that there are two marine terrace deposits are preserved in this location, and in this case, the younger one is resting on top of the older one (holding true to the Law of Superposition). This example show some complexity to the situation that may be related to nearby faulting along the Carmel Fault (discussed below).
Figure 88 is a close-up view of a weathering conglomerate bed, the rounded beach cobbles and gravel clasts are held together by in a poorly consolidated sandy/clay matrix that easily breaks apart. Figure 89 shows a close-up of a coarse sand bed in the Lindavista formation that displays small dark, round circles scattered across the surface. These are small ironstone concretions that have formed by wetting and drying, where the groundwater dissolves and redistributes the iron (in the form of limonite) to form the concretions. Some of the concretions preserve concentric growth ring (similar to those in a pearl or growth rings visible in a cut log). Because the ironstone concretions are more resistant to weathering and erosion they tend to get concentrated in the regolith or residual soil in many places where the Lindavista Formation crops out at the surface (Figures 90, 91, and 92).
The sedimentary layers in the marine terrace deposits display a variety of sedimentary structures (similar to those in the Eocene formations) including cross-bedding, contorted bedding, and sorting by grain size. The sedimentary layers display evidence of wetting and drying, forming large desiccation fractures and clastic dikes (Figure 93). Clastic dikes may form initially from deep desiccation fissure or tectonic fractures but then may be locally filled in with younger sand or mud that may have flowed in from above or had been injected upward from below by water pressure. The clastic dikes are usually a different color than the older, redder host sediments.
|
Fig. 82. View looking west from Torrey Pines Northern Extension to Torrey Pines mesa with Lindavista Formation cap rock visible along the skyline. |
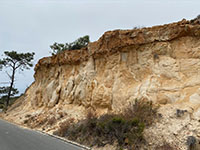
Fig. 83. Lindavista Formation forms the red cap rock on an eroded surface of the Clairemont marine terrace on Torrey Sandstone at the High Point Overlook area. |
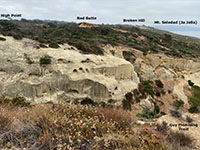
Fig. 84. View looking south from the Guy Fleming Trail with Red Butte and Broken Hill in the distance. Both red cap rock features are rest on the Clairemont marine terrace. |
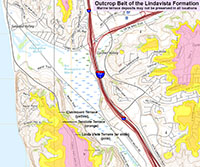
Fig. 85. Map of Lindavista Vista Formation's marine terrace belts in the Torrey Pines park area based on elevation data. |
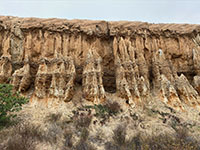
Fig. 86. Gravel and sand beds in the Lindavista Formation are well exposed along Torrey Pines Road (Old Coast Highway). |
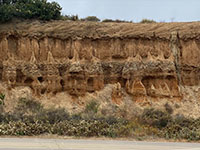
Fig. 87. This view shows one marine terrace deposit (with gravel and sand beds) repeated on top of another along the Torrey Pines Road grade. |
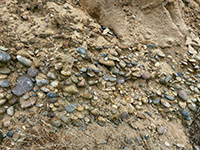
Fig. 88. Close-up view of a bed of poorly consolidated conglomerate (beach pebbles/cobbles) in the Lindavista Formation. |
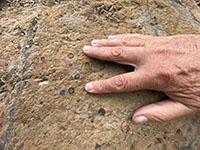
Fig. 89. This is a close-up view showing how ironstone concretions formed in place in some sandstone bed in the Lindavista Formation. |
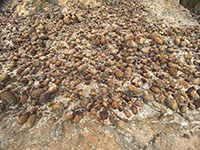
Fig. 90. Sandstone concretions weathered from the Lindavista Formation are concentrated in regolith/soil as loose sand washes away. |
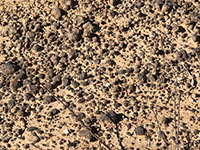
Fig. 91. Pebble-sized concretions are concentrated is the weathering regolith (soil) forming on the Lindavista Formation. |
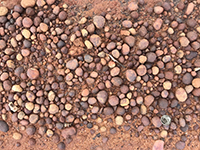
Fig. 92. More rounded ironstone concretions are abundant in some location, these are along the Northern Extension trails area |
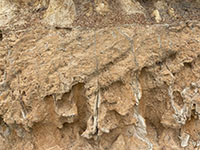
Fig. 93. Weathering along fractures and desiccation cracks forms clastic dikes in some sandstone beds of the Lindavista Formation. |
|
A17
Bay Point Formation
The Bay Point Formation is basically a younger version of the Lindavista Formation. It formed from deposits on a series marine terraces that formed in the late Pleistocene Epoch. However, the Bay Point Formation has not endured the higher degree of weathering alteration and erosion as the older Lindavista Formation.
Marine Terraces of the Bay Point Formation
Figure 94 is a map of showing the elevation belts where Bay Point Formation may occur based on elevation data (it may not be present or preserved in these locations). The name marine terraces associated with the Bay Point Formation that are recognized in the Torrey Pines park area include the Parry Grove, Guy Fleming, and Nestor marine terraces (see Figure 79). Of these tree, the Nestor terrace is easiest to see where it is exposed also the sea cliffs (Figures 95) and lower canyons (Figures 96 and 97). Figure 98 shows a close-up view of the basal layer of the Nestor marine terrace deposit showing a mix of cobbles, gravel, sand, and shell fragments. In the older terrace deposits (as in the Lindavista Formation) most of the shell material has dissolved away long ago.
The Nestor terrace formed as sea level approached a level that is about 20 feet higher today during the peak of the last interglacial warm period, about 120,000 years ago. The Nestor terrace now averages about 72 feet above current sea level. This shows that the rate of uplift along the coast average about 1/2 inch per century. Because the Nestor terrace is the youngest, it is well preserved and can be easily followed for long distance along the California coastline. The older Guy Fleming and Parry Grove terrace deposits locally occur higher on the seacliffs slopes and generally appear lighter in color than the Nestor terrace deposits (Figures 99 and 100).
The study of marine terraces is used to evaluate variations in regional uplift along the coastline, and to determine the relative rate of displacement along some faults that cut through the terrace, such as the Rose Canyon Fault in La Jolla. |
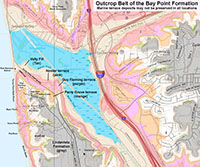
Fig. 94. Map of Bay Point Formation's marine terrace belts in the Torrey Pines park area based on elevation data. The colors show the contour intervals for the terraces where they may found if they haven't eroded away or not exposed. |
A18
Faults and Tectonism in the Torrey Pines Park Area
Regional tectonic processes are slowly shaping the landscape in the Torrey Pines region. Tectonism is responsible for the slow rise of the coastal region that has resulted in the formation of the marine terraces. That rise is both partly gradual, but may have also occurred suddenly during significant earthquakes of the past (none historically significant). Mueller [2002] calculated that some strata along parts of the San Diego coastline have undergone about 95 meters (<300 feet) of uplift since they were deposited in the middle to late Pliocene.
Features that tectonism created on a local scale that can be observed on the surface include folds and faults (Figure 101). Figures 102 and 103 are geologic maps (regional and local scales) that shows the general location of faults in the Torrey Pines park area. (Figures 1 and 6 also show mapped fault locations in the park area). Other large faults may exist in the area, but they are not exposed at the surface. Faults have long histories and may go through periods of inactivity, or can change with the interactions of other active faults. Long, straight valleys, especially those that cut across flat-lying sedimentary formations are often an indication of a fault. Because rocks along fault zones are typically highly fractured they are selectively likely to weather and erode faster, forming canyons and valleys. The nearby valleys (canyons) of the Los Peñasquitos Creek, Carmel Creek, and Sorrento Creek in the area have linear trends and likely follow fault zones. The location of Peñasquitos Lagoon is part of the linear trends that can be seen on satellite imagery geologic maps (see Figures 1 and 102).
Although there are no "major" faults in the park, several small faults to cut through the Torrey Pines area. The largest fault nearby is the Rose Canyon Fault. The Rose Canyon Fault is considered a major earthquake fault. The fault cuts through San Diego Bay, then runs offshore at La Jolla Cove. From there it continues northward along the continental shelf margin along the San Diego coastline—it occurs offshore of Torrey Pines park area (see geologic maps Figures 102 and 103). It merges along with other faults and continues up to the Los Angeles region.
The smaller Carmel Valley Fault cuts through the Torrey Pines mesa. It can be clearly seen in the road cuts along the Torrey Pines Road grade (Figures 104, 105, and 106). The road cut exposure show that erosion carved small canyons that back-filled with marine-terrace deposits, and these were later deformed by more-recent fault motion (Figures 107 and 108). The Carmel Valley fault zone crosses the Torrey Pines mesa and the fault zone expresses itself with offset faults, fractures, and folded beds exposed in the seacliffs along the northern part of Blacks Beach. Figures 109, 110, and 111 show views of the fault offsetting beds in the Torrey Sandstone. Another fault offsets beds of the Delmar Formation nearby (Figure 112). Also nearby is a fracture zone that may be part of the fault zone but does not display any vertical offset (Figure 113). Higher on the sea cliff at Blacks Beach the beds of the Ardath Formation appear warped in a complex anticlinal fold (Figures 114).
|
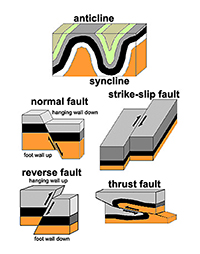
Fig. 101. Different kinds of folds and faults.
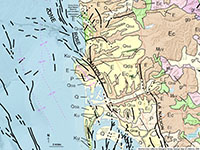
Fig. 102. Portion of the Geologic Map of California with Torrey Pines park area. The map shows rock formation ages, faults, and offshore bathymetry. |
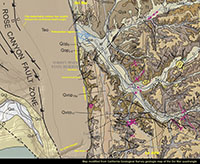
Fig. 103. Portion of the CSG Geologic Map of San Diego showing faults and structures in the Torrey Pines park area |
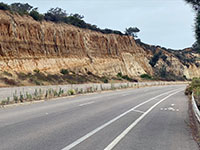
Fig. 104. View north along Torrey Pines Grade showing truncated beds along unconformities adjacent to the Carmel Fault Zone. |
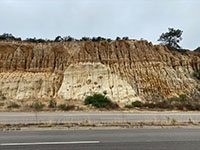
Fig. 105. The Carmel Fault Zone exposed along the Torrey Pines Grade offsets beds of the Torrey Sandstone and Lindavista Formation |
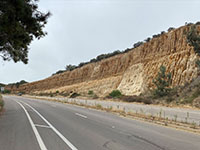
Fig. 106. View looking south at the Carmel Fault Zone along the Torrey Pines Grade. Offset of Pleistocene-age beds makes it an "earthquake fault." |
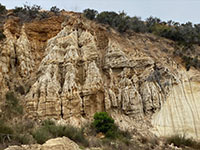
Fig. 107. Close-up view of fractures and warping offset of Pleistocene-age beds in the Carmel Fault Zone along the Torrey Pines Grade. |
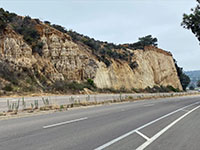
Fig. 108. View of the north side of the Carmel Fault Zone showing massive cliff of the Torrey Sandstone along the Torrey Pines Grade. |
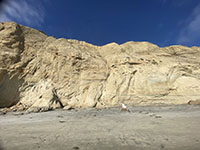
Fig. 109. Fault mapped as part of the Carmel Fault zone on Blacks Beach offsets beds of the Torrey Sandstone and Ardath Shale. |
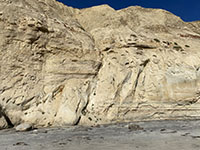
Fig. 110. A normal fault (Carmel Fault?) offsets massive blocks of Torrey Sandstone (down on the left) on Black Beach. |
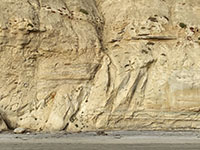
Fig. 111. Close-up view of the fault on Blacks Beach. It appears "normal" but may have a significant "strike-slip" component as well. |
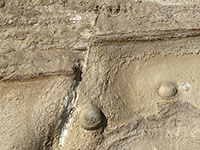
Fig. 112. This small normal fault offsets layers of sandstone (with concretions) in the Delmar Formation at Blacks Beach. |
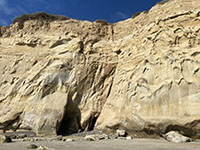
Fig. 113. Fractures in the Torrey Sandstone make spots like this on Blacks Beach subject to selective wave erosion forming sea caves. |
Fig. 114. Large anticlinal fold in the Ardath Shale is a complex feature with slump-like features offsetting beds of sandstone and mudrocks. |
|
A19
The coastline is a very dynamic environment. Day-to-day wave action and tides are constantly moving sediments. When a strong winter storm occurs with high tides, large volumes of sediments can move in a short period, stripping away vast amounts of beach sand and undercutting seacliffs to cause cliff failures.
Over time, sea level is rising (significantly faster than the land is rising).
The most recent glacial period peaked about 21,500 years ago during the Last Glacial Maximum (LGM). During the peak of the last ice age, California was very different than what we see today (Figure 115). An ice cap covered the Sierra Nevada Range and the high peaks in Northern California. Overall the climate was also much wetter. Large lakes flooded all the inland valleys in the Mojave region and elsewhere where the drainages did not reach to sea. At the LGM, sea level was almost 400 feet lower and shorelines were located at the edge of the world's continental shelves. In California, an extensive coastal plain extended offshore, many miles in some areas. For instance, the Channel Islands north of Los Angeles were connected to the mainland, and the coastline was nearly 50 miles west of where the Golden Gate Bridge is in San Francisco. In the San Diego area, the edge of the continental shelf is roughly a couple miles offshore. That portion of shelf would have been exposed as coastal plain (see Figures 6 and 102).
Perhaps what would be most remarkable about that time is that extensive forest communities extended across vast parts of California that is now treeless desert. The Torrey Pines coastal chaparral plant community (in Torrey Pines) was possibly connected with its relative populations that currently exist on Santa Rosa Island off the coast of Santa Barbara, California. A long list of large mammal species including mammoths, mastodons, saber-tooth tigers, dire wolves, and many others existed in the region, but have all gone extinct at the end of the last of the ice age.
The continental continental ice sheets began to melt in earnest about 14,000 years ago, but slowed significantly starting about 7,500 years ago. Sea-level rise slowed significantly to several inches a century—note that the world's ancient civilizations began to evolve at that time! Unfortunately, with modern climate change the rate sea level rise is significantly increasing again. As sea level has risen, it has caused coastal sedimentary processes of erosion and deposition to change and gradually shift landward as sea level rises. |
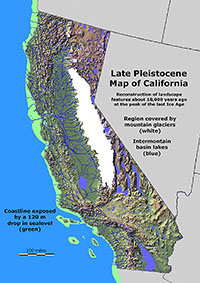
Fig. 115. Map of California at the peak of the last Ice Age. Glaciers covered the Sierra Nevada Range, large lakes filled internally-drained basins, a broad coastal plain extended seaward because sea level was lower, and forests covered many regions that are now barren desert. |
A20
Modern Deposition and Erosion Processes In the Torrey Pine Park Area |
Erosion and depositional process are actively shaping the landscape in the Torrey Pines park area producing surficial deposits and shoreline deposits.
Surficial Deposits included alluvium, colluvium, and soil. These terrestrial sediments eventually migrate to the lagoons, beaches, and offshore. Alluvial deposits include detrital materials deposited by stream flow and slopewash (from heavy precipitation). Alluvium is found underlying stream channels and floodplains, and consists mostly of sand and mud (a mix of sand, silt and clay). Beds of gravel are associated with channel deposits where strong currents winnow away finer sediments. Colluvium is unconsolidated materials derived from bedrock including blocks of material derived by creep or landsliding (small to large scale. (Landsliding is discussed below.) Colluvium is typically mixed with talus and slopewash sediments washed downslope by sheet flow from heavy precipitation. Soil forms on surfaces where plant communities become established and roots and organic detritus hinder surface erosion. Plants and bioturbation by burrowing organisms contribute decaying organic matter to the upper soil profile.
Shoreline deposits include sediments deposited in a variety of depositional settings (see Figure 9). Sand dominates beach and dune deposits and near-shore marine (littoral) bar and seafloor deposits. Wave action and currents sort sediments—coarser fractions (gravel and sand) can accumulate in higher energy settings (beaches and stream channels), whereas finer materials (silt, mud, clay) are carried away to quieter water settings (offshore or bays/lagoons).
Beach Dynamics: Beach sediments are transported by wave action, carried by longshore drift (forming beaches and bars), and moved by wind action (forming coastal dunes). The sources of sand and gravel comes from sea-cliff erosion and the occasional alluvial discharge from streams, and slopewash erosion and landsliding of surficial deposits. Beach deposits are composed primarily unconsolidated sand, and locally underlain by layers of gravel and cobbles. The thickness of beach deposits varies seasonally, typically less than 25 feet thick. Sand moves onto the beach from offshore sand bars in seasonably quiet weather of the summer but moves offshore again during the winter season when wave action increases. |
A21
Features Along Torrey Pines Beaches and Bluffs |
Figure 116 is a view looking south along Torrey Pines Beach. Torrey Pine Road causeway has replaced the natural bay-mouth bar that previously existed in the area. The mouth of
Peñasquitos Lagoon is now restricted by the causeway and bridge. Before the road and causeway the mouth the lagoon would have episodically shifted to other location. Figure 117 shows the ebb-tidal delta at the where outflow form the lagoon deposits sand. A sand bar (with runnel) near the mouth of the lagoon is exposed at low tide (Figure 118).
South of the lagoon the beach is constrained by the seacliffs (Figure 119). When high tides combine with strong wave action from storms the beach is completely submerged and waves crash against the base of the seacliffs. Strong wave action actually scours though the beach deposits carving away at the wave-cut platform on the surface of the bedrock. Figure 120 shows where a stream drainage intersects the sea cliff creating a dry waterfall. It shows that wave erosion is the dominant erosion force.
Figure 121 shows a beach berm established on a shingled (cobbled) part of the beach. The berm and the gravel are leftover features from the winter stormy season. A beach that is covered with sorted pebbles and gravel is called a shingle beach. Shingle beach features typical are well developed on the upper beach and form in the winter season. In the summer season they may be partly or fully buried beneath sand. Figure 122 show the well-sorted character of the beach gravel with most clasts in the 2-3 inch diameter range. Figure 123 shows a collection of gravel clasts sorted in somewhat of a color wheel fashion. The rounded clasts on the beach are durable cobbles reworked from the older rock formations along the coast, most likely reworked from the ancient Ballena River alluvial deposits that were carried here from volcanic source areas in the Sonora region of Mexico. Some of the clasts also are probably from the local mountain sources; some clasts bear resemblance to clasts found in river terrace deposits along the southern Colorado River drainage.
Figure 124 shows a portion of the beach near Flat Rock that was entirely covered with black sand in the winter season. The black sand is mostly magnetite, a common durable, dense iron-oxide mineral derived from granitic source rocks—the dominant rock type in Peninsular Ranges of San Diego County. The dense magnetite sand is concentrated by wave action that sorts and removes the lighter quartz sand.
Figures 125, 126, and 127 show Flat Rock, a prominent feature at the point of the headlands between Torrey Pine Beach and Blacks Beach (to the south). Flat Rock is a wave-cut bench which is an elevated remnant of the wave-cut platform. Wave-cut benches can be indicators of past sudden uplift from major earthquakes along a coastline. Flat Rock is an eroded remnant of a hard flat-lying sandstone layer of the Delmar Formation. It can also be considered a sea stack because it is a mass of rock detached by wave action from a cliff-lined shore and surrounded by water. Figure 105 shows a water-filled, square-shaped pit that was cut into the surface Flat Rock. Local lore is that this was an exploratory shaft that was dug in a failed attempt to find a coal bed in the Delmar Formation below the sea bed to possibly supply coal for a proposed railroad. The railroad was eventually constructed without a supply of local coal.
Figures 128 shows another wave-cut bench near Flat Rock and how wave action is undercutting the sea cliffs in Torrey Sandstone on Blacks Beach. Figure 129 shows large boulders sculpted by wave action beneath the overhang in the cliff. Strong wave action during storm hurls hard cobbles and loads of sand against the base of the seacliffs. This abrasive action undercut the sea cliff leading to cliff failures. Along fractures and zones of weakness allow for the formation of sea caves (Figure 130).
Figure 131 shows how differential erosion affects the shoreline with this view looking north at Blacks Beach from the Torrey Pines Gliderport. The headlands near Flat Rock at the north end of Blacks Beach is underlain by the more erosion-resistant sandstone and marl beds of the Delmar Formation, whereas the wider part of the beach is under and adjacent to the softer Ardath Shale. As a result wave action over time is carving the wave-cut platform and seacliff faster on Blacks Beach compared to the headlands area.
|
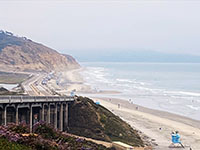
Fig. 116. Zoomed-in view of the beach at low tide with ebb-tidal delta bar features visible. The bay-mouth bar is now a Torrey Pines Road causeway. |
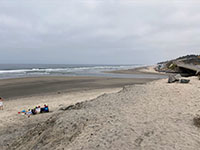
Fig. 117. Ebb tidal delta exposed at low tide at the mouth of the Peñasquitos Lagoon. Its location is limited by the bridge. |
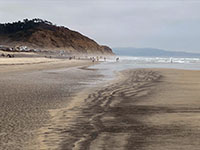
Fig. 118. Sand bar and runnel exposed at low tide at the south side of the tidal inlet on Torrey Pines Beach.
|
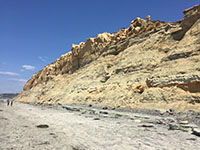
Fig. 119. View of the massive seacliff along Torrey Pines State Beach. The beach sand rests on the wave-cut platform at the base of the sea cliff. |
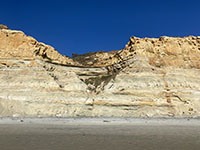
Fig. 120. Dry waterfall and alcove in the seacliff in the stream drainage between Razor Point and Perry Grove Trail. |
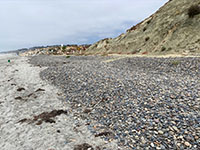
Fig. 121. Winter wave-generated beach berms , shingled gravel bars adjacent to the sea cliff at Torrey Pines Beach. |
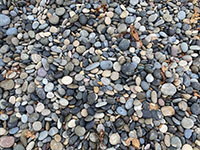
Fig. 122. Wave-polished pebbles and cobbles of a shingled beach deposit on Torrey Pines State Beach
|
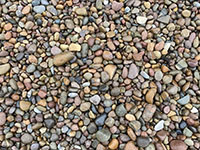
Fig. 123. Gravel from the beach at the south end of Blacks Beach are derived from the Scripps Formation.
|
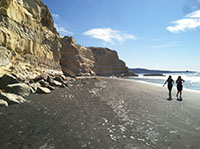
Fig. 124. Black sand (mostly magnetite) is concentrated on the beach by wave winnowing action mostly in winter months. |
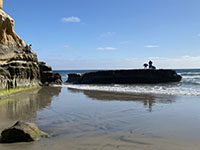
Fig.125. Flat Rock is a prominent feature dividing Torrey Pines Beach (to the north) and Blacks Beach (to the south). |
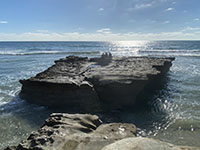
Fig. 126. Flat Rock is both a wave-cut bench and a sea stack (when it is separated from the shoreline).
|
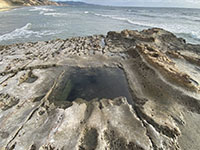
Fig. 127. A square water-filled pit on Flat Rock was an unsuccessful attempt to find a coal bed layer in the Delmar Formation. |
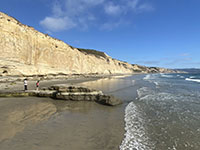
Fig. 128. This view looking south from Flat Rock at Blacks Beach massive seacliff of Torrey Sandstone with a wave-cut-bench and beach. |
Fig. 129. Storm and high-tide wave action tosses gravel and rock fragments, undercutting the base of the seacliff, creating a cliff failure hazard. |
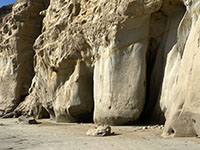
Fig. 130. Sea caves selectively carved by wave action along fracture zones in the Torrey Sandstone on Blacks Beach.
|
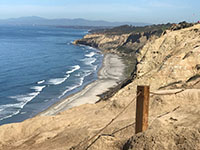
Fig. 131. Blacks Beach at the south end of Torrey Pines Park. The headland in the distance is at Flat Rock north of the Carmel Fault zone. |
|
A22
Sea Cliff Landsliding Features and Hazards In the Torrey Pines Park Area
Sea cliff failures kill and injure people every year in California, and the beach/sea cliffs in the Torrey Pines area has had its share over the years. Sea cliff rock-fall warning signs are posted along the beach (Figure 132). Coastal erosion and sea cliff collapse is a HUGE problem in San Diego and coastal erosion specialist mention the heavily developed San Diego coastline north of Torrey Pines frequently in their discussions and research. The rate of coastal erosion varies from place to place and from year to year. Beach sand erosion is easiest to see from year to year. "California’s coastal erosion rates generally range from 0-42cm/year; however, specific sites can retreat at much higher rates." (Beachapedia, 2018).
Sea cliff failures involve a variety of landsliding: including topples, rock falls, debris flows, creep, and slumps (Figure 133). The US Geological Survey defines a landslide as "the movement of a mass of rock, debris, or earth down a slope. Landslides are a type of 'mass wasting,' which denotes any down-slope movement of soil and rock under the direct influence of gravity."
Figures 134, 135, and 136 illustrate seacliff failures along Torrey Pine Beach (recent in August, 2020). Rockfalls create talus cones at the base of the sea cliff. In some cases, tumbling blocks can roll many meters from the sea cliff. A general guideline is stay at least 20 feet away from the seacliff (or more).
Cliff failures can be massive! Figure 137 illustrates a cross-section showing features of a massive coastal slump. Blacks Beach is host to an example of one, shown in Figures 138 and 139. Massive slumps like this one are typically slow moving. Wave erosion carves away at the toe of the slump, revealing highly contorted bedding exposed in tide pool outcrops along the beach.
|
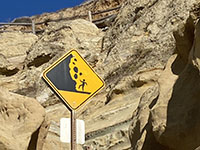
Fig. 132. Signs with the universal symbol for rock-fall hazards along seacliffs are posted warnings in the park. |
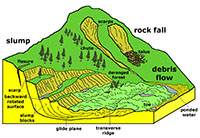
Fig. 133. Different kinds of landslides and landslide features. |
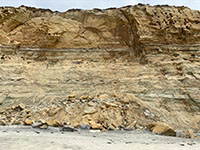
Fig. 134. Rock-fall material (talus) accumulates at the base of the seacliff at Torrey Pines Beach. |
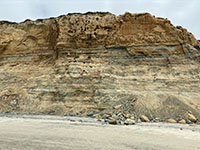
Fig. 135. Rockfall material accumulates at the base of the seacliff. Rock falls are a common occurrence. |
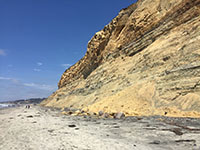
Fig. 136. A talus cone created by slowly accumulating finer debris at the base of the seacliff on Torrey Pines Beach. |
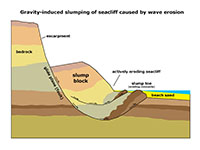
Fig. 137. Diagram showing a cross section of a massive slump caused by seacliff failure along a beach. |
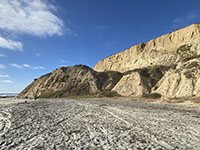
Fig. 138. View looking north at the south end of a massive slump block on Blacks Beach.
|
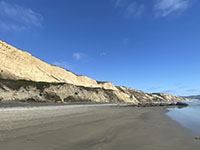
Fig. 139. View looking south at the north end of the massive slump block on Blacks Beach.
|
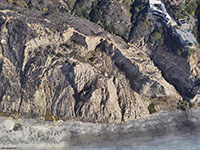
Fig. 140. A massive slump (landslide) forming in the sea cliff north the Sauk Institute Beach Trail offset Ardath Shale and Scripps Formation. |
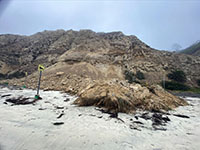
Fig. 141. A massive cliff failure (rock fall/landslide) extends for more than 100 feet onto Blacks Beach. Note trees in the toe closest to camera. |
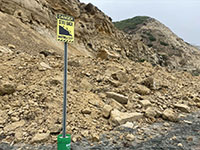
Fig. 142. Close-up view of the massive rock fall deposit on Blacks Beach. The large block on top is about 10 feet in diameter. |
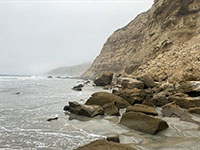
Fig. 143. Massive blocks of rock cover the narrow beach below the outcrops of the Scripps Formation exposed in the sea cliff near Dike Rock. |
|
A23
Peñasquitos Lagoon
Figure 144 shows a satellite view of Peñasquitos Lagoon. The lagoon consists of a mix of salt marsh, tidal mudflats, and tidal channels protected within the Los Peñasquitos Marsh Natural Preserve. The lagoon is host to a Coastal Strand plant community. Plants in the marsh are capable of enduring exposure to salt-laden water. Sunlight and daily tidal flushing provides nutrients to support algae, plankton, and plant growth that feeds and shelters crabs, fishes, clams, mussels, oysters, shrimps, snails, and worms that dwell in the salt marsh and tidal channels. These, in turn, feed shorebirds, waterfowl, rays, and other larger fishes (and until recent times, Indians and early settlers that harvested food from the lagoon).
The ancient peoples that were in the San Diego region about 12-10,000 years ago had a very different view of the Torrey Pines park area.
At that time the valley occupied by Peñasquitos Lagoon would have been a canyon possibly a couple hundred feet deeper, and Peñasquitos Creek would have flowed westward from the current shoreline a couple miles across a low coastal plain to a beach/shoreline (where the continental shelf margin exists offshore today). As the last ice age ended and sea level began to rise rapidly, the Peñasquitos Creek canyon gradually evolved into a bay along the coastline, probably with a nice sandy beach. As sea level continued to rise, the modern sea cliffs began to form and retreat landward and sediments began filling the bay. Eventually, a bay-mouth bar developed. The bay-mouth bar was basically a narrow barrier island with a beach and coastal dunes with a shifting inlet that allowed tidal currents to move sediments into and out of the ever expanding lagoon.
Figures 145, 146, and 147 show views of Peñasquitos Lagoon and surrounding upland mesas. The modern lagoon is mostly filled in with sediments but experiences daily flood tides and occasional storm surge flooding. As sea level continues to rise, the lagoon filling will keep pace. Figure 148 illustrates how lagoons gradually form and vanish as a result of sea level changes associated with the ice ages. In some locations, the surface of older lagoon deposits are preserved in step-like fashion along the margins of the modern lagoon (in similar character to the step-like nature of the marine terraces of the Bay Point Formation). Figure 149 illustrates a step-like feature that looks like a previous-cycle bay filling deposit (possibly modified with additional fill from the Torrey Pines Road grade construction). Step-like stream terraces also occur along upland stream and river valleys. The rise and fall of sea level changes the stream gradient so the streams go through cycles where they are either filling in and broadening their valleys, or down-carving—incising into their flood plains when their stream gradient increases.
Figures 150, 151, and 152 show the lower main channel area of
Peñasquitos Lagoon on the east side of the Torrey Pines Road causeway bridge. Incoming flood tides carry sediments from the beach side of the causeway and selectively dump the coarser gravel and sand, creating a flood-tide delta. The finer sediments are carried inland and are deposited on tidal flats or trapped by dense plant grow on the tidal marshlands. The sandy deposits on the flood-tide delta are popular roosting and nesting sites for shore birds. However, every few years now construction crews bulldoze these deposits out to replenish sand to the beach.
Figures 153, 154, and 155 show the ebb-tide delta area at the mouth of
Peñasquitos Lagoon on the west side of the Torrey Pines Road causeway bridge. Sediment supply and seasonal wave action greatly impacts this portion of Torrey Pines Beach. Figure 153 shows the area during the peak of the winter season when during high tide the sandy beach is entirely missing and a narrow shingle gravel deposit is a that remains. Figures 154 and 155 shows the same section of the beach during the summer when calmer wave activity allows sand to accumulate on the beach and shallow sand bars around the mouth of the lagoon. |
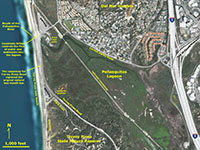
Fig. 144. Satellite map of the Peñasquitos Lagoon area. Torrey Pines Road causeway replace the natural bay-mouth bar that existed before the road
|
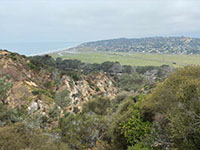
Fig. 145. View of Peñasquitos Lagoon from along the Torrey Pines Road grade (looking north). The lagoon is green in the winter months. |
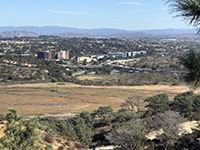
Fig. 146. View of the eastern end of Peñasquitos Lagoon from the park Visitor Center overlook. The lagoon turns brown in the late summer. |
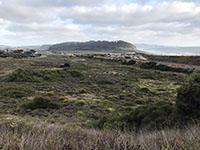
Fig. 147. View looking south at Peñasquitos Lagoon and the Torrey Pines mesa from Carmel Valley Road
|
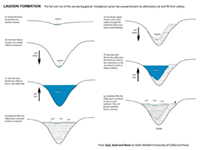
Fig. 148. This diagram shows how the fall and rise of sea level during ice ages carve canyons and then back fills them, forming lagoons.
|
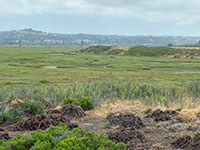
Fig. 149. A low terrace of valley-fill deposits on the south side of Peñasquitos Lagoon near Torrey Pines Road grade located along the Shore Trail. |
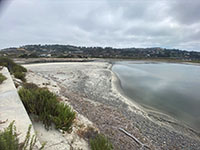
Fig. 150. Flood tidal deposits on the southeast side of the Torrey Pines Road bridge (main channel) in Peñasquitos Lagoon.
|
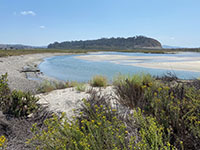
Fig 151. Sand bars on the flood tide delta deposits on the inland side of the inlet near the Torrey Pines Road bridge near the mouth of the lagoon.
|
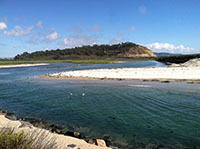
Fig. 152. Sand and gravel deposit on the flood-tidal delta in Peñasquitos Lagoon on the side of the Torrey Pines Road causeway bridge. |
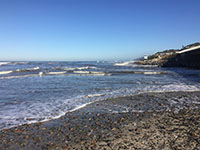
Fig. 153. Mouth of the Peñasquitos River in the winter when most of the sand has eroded away, leaving gravel behind. |
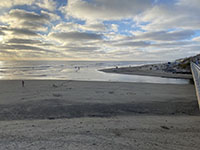
Fig. 154. Peñasquitos ebb tidal delta in the summer. Its location is controlled by the location of the causeway bridge of Torrey Pines Road. |
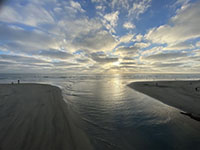
Fig. 155. The channel of the mouth of the Peñasquitos Creek (lagoon) where it drains into the Pacific Ocean (shown here at low tide). |
|
A24
Human Impacts
Thanks to the historic efforts of the founders of the Torrey Pines State Nature Preserve, the land within the park area is protected from further development. Unfortunately, human activity had significantly modified the landscape.
Humans well back into prehistory have been utilizing the Torrey Pines area. Humans may have settled in the coastal San Diego area as early as 20,000 years ago (San Diego History Center, 2020). Traces of Indian shell middens can still be found in some locations along the shoreline of Peñasquitos Lagoon. It is estimated that there were about 20,000 native people in the San Diego region about the time Spanish explorer Juan Rodriguez Cabrillo arrived and claimed San Diego area for Spain in 1542. That population crashed at the expense of pandemics and warfare as the Spanish migrated into the San Diego region. The first significant impact was the development of the El Camino Real (Kings Highway) along the eastern shore of Peñasquitos Lagoon.
San Diego came under Mexican rule for about 25 years starting in 1821. Mexican occupation ended with the conclusion of the war between Mexico and the United States in 1848 just prior to the California Gold Rush of 1849. The first census of "non-Indian" population of San Diego was 650 people in 1850.
The first major "modern" impact that affected the Torrey Pines park area was the construction of the Southern Pacific Railroad line. The section between San Diego and Temecula was completed in 1881. Prior to that time the park area was basically under-utilized except for ranching (cattle and sheep)and woodcutting. Torrey Pines was first established as a public park in 1889 (see History of the Reserve by the Torrey Pines Docent Society).
|
Fig. 156. The U.S. Geological Survey La Jolla 15 minute topographic map completed in 1903. Even in 1903, the Torrey Pine park area remained largely undeveloped. |
Figure 156 shows the U.S. Geological Survey La Jolla 15 minute quadrangle topographic map completed in 1903. The map show that the Torrey Pines park area was still largely undeveloped. The map shows precursor of the Torrey Pines Park Road crossing the mesa area and then winding back along the south shore of the lagoon to merge with the El Camino Real along the east end of Peñasquitos Lagoon. Carmel Valley Road ran along the north shore of the lagoon connecting the El Camino Real with the small train depot town of Del Mar. The map also shows the original railroad causeway cutting across the east end of the lagoon. The 1903 map shows that there were two inlets cutting across the Torrey Pines Beach bay-mouth bar along the coast and that those channels had already been modified by dredging.
After World War 1, the population of the San Diego region began to increase significantly. The first major construction project to harm the natural setting of of the park area was the construction of the Santa Fe Railroad causeway and bridge. It was first construction in 1925 (with new a bridge and causeway modifications since then, Figure 157). This lead to the next large construction project-the construction of the Pacific Coast Highway. The Torrey Pines Park Road was the original route of the Pacific Coast Highway ((the Park Road now begins at the park entrance, Figure 158). Construction of the new route of the Pacific Coast Highway began in the early 1930s. This lead to the excavation of the what is now Torrey Pines Road causeway and bridge (Figures 159 and 160) and the road cuts for the Torrey Pines Road grade. A new causeway for the railroad line cutting across Peñasquitos Lagoon
The designation of the Coast Highway in the Torrey Pines area ended with the largest construction project, the construction of Interstate 5. Construction of I-5 began in 1947 and was first completed in 1967, but construction never really ended. If you look at the current satellite view of the complex highway system between the intersections of I-5, I-805, and the Ted William Freeway (CA 56) you can count as many as 30 lanes for traffic! It's hard to envision what the future will hold. The San Diego population will probably eventually stabilize, the carbon-based fuel economy will diminish. However, sea level will continue to rise, sea cliffs and beaches will erode, and the climate will continue to change. The need for conservation efforts will only increase. |
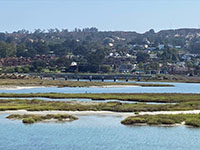
Fig. 157. The Santa Fe Railroad causeway and bridge across Peñasquitos Creek in the lagoon was constructed in 1925. |
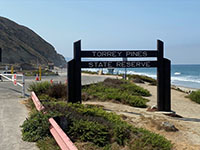
Fig. 158. Entrance sign to Reserve in the Park Road. The Park Road was the original Pacific Coast Highway.
|
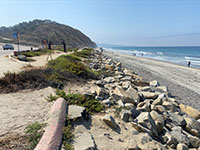
Fig. 159. The Torrey Pines Road Causeway is protected by rip-rap construction boulders to withstand wave erosion. |
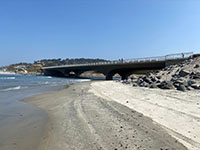
Fig. 160. The modern Torrey Pines Road causeway bridge over Peñasquitos Creek.
|
|
Torrey Pines Extension
The Torrey Pines Extension is an isolated northern part of the Torrey Pines State Nature Reserve. Figure 161 is a map of the Extension. Figure 162 is a satellite image of the same area in Figure 161. The Extension is located along the southern part of the mesa of the Del Mar Heights Community. Several hiking trails provide access to this scenic less-traveled part of the Torrey Pines State Nature Reserve.
The Torrey Pine Extension hiking trails are accessible from trailheads in several locations around the reserve. An easy place to park is the vicinity of Del Mar Heights Elementary School on Mira Montana Drive. Both the Red Ridge Trail and Margaret Fleming Nature Trail start at the end of Mira Montana Drive.
Another trailhead is at the north end of Del Mar Scenic Parkway that connects to the Margaret Fleming Nature Trail and the Mar Scenic Trail.
The landscape consists of rolling hills along an south- and west-facing escarpment with ridges that extend southward into a landscape dominated by a mixed coastal sagebrush and coastal oak plant community that is also host to small groves and isolated Torrey Pines (the endangered pine found in the area)(Figures 163 to 168). |
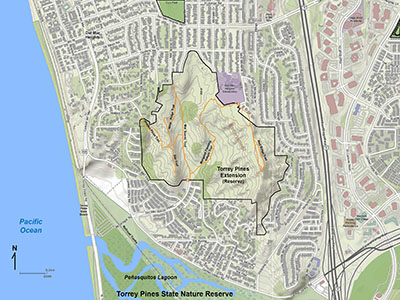
Fig. 161. Map of the Torrey Pines Extension, the northern part of the Torrey Pines State Nature Reserve.
|
Geology of the Torrey Pines Extension Area
Figure 169 is a generalize geologic cross section of the Extension area (see Torrey Pines Stratigraphy [Figure 7]). The geology is very similar to the Torrey Pines Mesa area.
The Delmar Formation (Middle Eocene age) exists beneath the lower slope areas near the lagoon by is not exposed below plant cover and surficial colluvium deposits.
The Torrey Sandstone (Middle Eocene age) is locally exposed as massive white outcrops of cross-bedded sandstone locally in the middle slopes and along ridges (Figures 170 to 172).
The Lindavista Formation (late-Early Pleistocene) unconformably overlies the top of the Torrey Sandstone and forms the orange-brown caprock surface of the surrounding mesa (Figures 173-179). It includes the soft, badlands-forming sediment beneath the caprock surface. The Linda Vista Formation is locally about 20-30 feet thick. The top of the mesa is locally covered in extensive areas by small ironstone concretions (most are roughly 0.5 to 2 inches in diameter). The concretions are accumulating on the surface as softer, cement-depleted sandstone erodes away, concentrating the tightly cemented, sandy ironstone concretions on the surface (Figures 180 and 181). The ironstone concretions may have formed where pyrite/marcasite (FeS2) crystals have been replace through leaching and oxidation by hematite (Fe3O4), limonite (FeO(OH)·nH2O), and other metal oxides, creating the dense matrix holding the concretions together. The pyrite probably formed in the organic-rich silt/sandy sediments in a marine setting when sediments of the Lindavista Formation were originally deposited in early-Late Pleistocene time when the marine terraces associated with the formation were actively forming. As the land rose, and old marine terraces became exposed to sub-aerial weathering. The iron-oxide minerals are more stable than other minerals in arid and semiarid settings. Ironstone concretions can be found in association with the oldest marine terrace deposits in many locations through San Diego County.
The Bay Point Formation (Late Pleistocene) occurs in scattered pockets on the slopes between the the Linda Vista escarpment and the lagoon. The marine terrace deposits and features along the coast transition to lagoonal shoreline deposits and elevated stream terrace deposits and features along Sorrento Valley (and its stream tributary valleys). The ancient terraces are preserved as step-like benches in some places along the canyons, but most have degraded or eroded away over time. Evidence of the remnants of Bay Point Formation can be seen in the form of patches of sorted gravels in some areas along the trails (Figures 182 and 183). These gravels were originally derived and reworked from older Eocene-age river deposits and reworked by stream and wave action as sea level rose and fell during the Pleistocene ice ages. Figure 184 is a view from Red Ridge that shows a local outcrop of the Bay Point Formation (gray) below outcrops of theLindavista Formation (reddish) and on top of Torrey Sandstone (white).
Mesa lands and mountain peaks east of Torrey Pines Extension: Figure 185 provides a panoramic view looking to the east of Red Ridge in the Torrey Pines Extension area. The view shows the broad mesa that extends to the base of the foothills, highlands, and mountain peaks in the core of the Peninsular Ranges. The mesa top extends east across the Miramar Mesa region and is capped by the Lindavista Formation of Early Pleistocene age (and possibly older) when the mesa top was actually the eroded surface of the shallow continental shelf and embayments when the land was 400 to 800 feet lower than today (and/or sea level was also higher). The ancient shoreline ran along the base of the mountain front. Since then, the mesas area has been uplifted and dissected by streams that carved canyon. Some of the canyons follow carved zones of weakness in the rocks along fault zones that cut the mesas. |
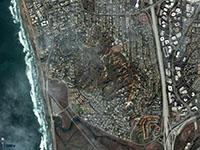
Fig. 162. Satellite map view of the Torrey Pines Extension area (same as the map area shown in Figure 161). |
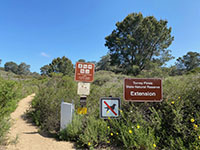
Fig. 163. Trailhead at the end of Del Mar Scenic Parkway on the south side of the Extension. |
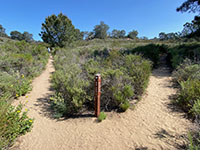
Fig. 164. Intersection of the Margaret Fleming Trail and the Mar Scenic Trail in Torrey Pines Extension.
|
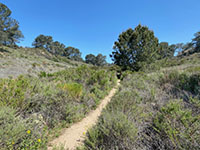
Fig. 165. View of Torrey Pines and coastal sage plant community along the Margaret Fleming Trail.
|
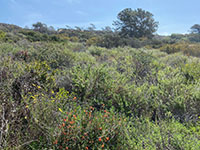
Fig. 166. Coastal sagebrush plant community in valley below escarpment.
|
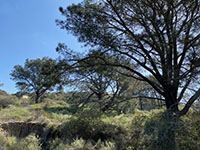
Fig. 167. Torrey Pines on the mesa top in the Extension of Torrey Pines State Nature Reserve.
|
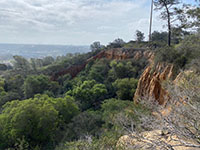
Fig. 168. Coastal oak grove below escarpment of Lindavista Formation.
|
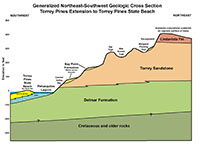
Fig. 169. Generalized geologic cross section: Torrey Pines Extension (east) to the Torrey Pines State Beach (west).
|
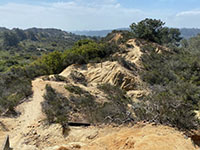
Fig. 170. Ridge of erosional resistant Torrey Sandstone is probably bounded by valleys along eroded fracture zones.
|
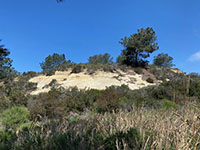
Fig. 171. Massive cross-bedded layers in the upper Torrey Sandstone Formation.
|
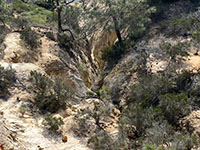
Fig. 172. A narrow slot canyon eroded into the Torrey Sandstone (inaccessible for hiking). |
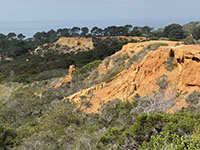
Fig. 173. View looking west along the Lindavista caprock escarpment.
|
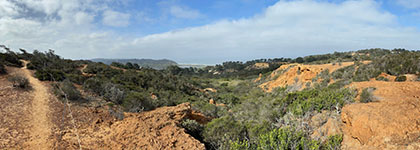
Fig. 174. Panoramic view from the Lindavista caprock escarpment looking down the canyon along the Margaret Fleming Nature Trail with the lagoon in the distance. |
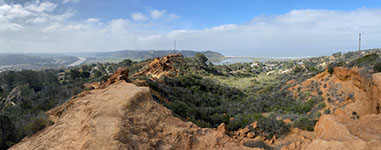
Fig. 175. Panoramic view from along the Lindavista caprock escarpment looking down the Red Ridge Trail with Interstate 5 in Sorrento Valley (distant left). |
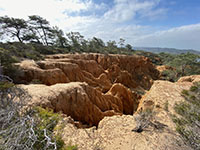
Fig. 176. Badlands eroding in the the poorly consolidated sediments below the tightly cemented caprock of the Lindavista Formation. |
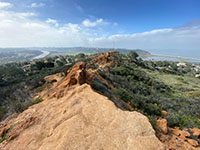
Fig. 177. View south along the Red Ridge Trail with pinnacles of Lindavista caprock: Torrey Pines Mesa, lagoon, and I-5 in distance. |
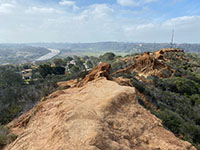
Fig. 178. Zoom view with Red Ridge with upper lagoon in Sorrento Valley and I-5 north of La Jolla and Rose Canyon corridor. |
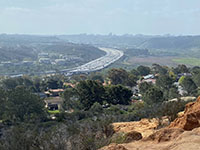
Fig. 179. Combination of Interstate 5, I-805, and Highway 52 results in 20 traffic lanes in Sorrento Valley on east side of the lagoon. |
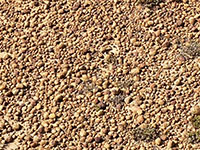
Fig. 180. Ironstone concretions concentrated on the surface by differential erosion of softer sandy sediment. |
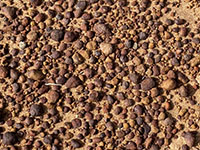
Fig. 181. Hematite and other iron mineral cement gives the sandy ironstone concretions their hard, dark brown appearance. |
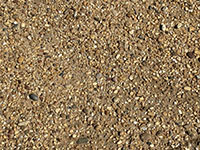
Fig. 182. Example of a well sorted fine gravel of the Bay Point Formation that is dominated by white quartz pebbles. |
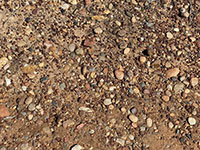
Fig. 183. Coarser gravel bed in the Bay Point Formation. Gravel is reworked from older formations into marine terrace and stream deposits.
|
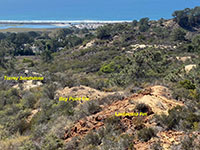
Fig. 184. View near Red Ridge of a Bay Point Formation outcrop. |
Fig. 185. Panoramic view looking east (from north to south) of the mesa region east of the Torrey Pines Extension with the distant peaks labeled (to be revised). |
|
North Beach (Torrey Pines State Beach)
North Beach is about a mile-long section of beach and sea cliffs north of the
bridge over the tidal inlet to Peñasquitos Lagoon (Figure 186). This section of the state park beach is somewhat less visited than the park beach south of the bridge. The setting isn't quite as scenic or natural as the southern part of the park, partly because the coastal rail line cuts along a man-modified shelf on the sea cliff (Figures 187 and 188). In addition, a Delmar Heights housing development caps the northern end of the sea cliff, and part of the sea cliff is currently being modified with a sea wall to prevent erosion and landsliding impacting the rail line (Figure 189). However, because of the rail line and sea cliff, the beach is less accessible, and is therefore generally less traveled (Figures 190-192). North of the state park boundary the public beach is part of Del Mar South Beach Park (Figure 193).
Sedimentary rocks exposed in the lower part of the sea cliff are part of the Del Mar Formation (Middle Eocene)(Figures 194 to 196). The sedimentary deposits consist of poorly consolidated, silty green shale with lighter-colored small to larger lens-shaped sand bodies that represents ancient submarine channel fill deposits. Figure 197 shows a series of clastic dikes extending downward from the base of a channel sandstone deposit. Features like this suggest that the underlying mudrock may have been experience subaerial exposure before sea level rose again and shallow marine channel sediment fill was deposited on top.
Near the north end of the Torrey Pine Park section of the beach a small fault is partially exposed in the sea cliff. This is adjacent to a pipe where spring water drains onto the beach (Figures 198 and 199). The fault only shows about a meter of vertical offset that cuts across the base of the Nestor marine terrace deposit (orange in Figure 198). Although is is probably a minor fault ore the top of a glide plain in a slump, it shows that the coastline is geologically active.
|
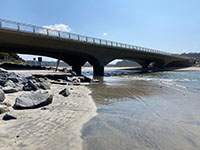
Fig. 186. Bridge over the tidal inlet to Los Penasquitos Lagoon. North Beach extends north of the bridge about a mile to Delmar South Beach.
|
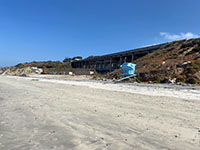
Fig. 187. View looking north along Torrey Pines State Beach, North Beach toward the rail line causeway-overpass bridge.
|
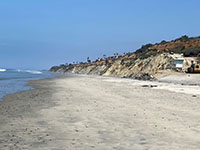
Fig 188. View looking north along the North Beach. The rail line runs along a bench on top of the Del Mar Formation and below the Bay Point Formation. |
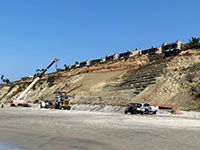
Fig 189. Construction of a sea wall is intended to protect the coastal rail line that runs along the top of the Delmar Formation from erosion and landsliding.
|
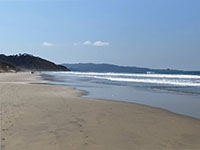
Fig 190. View looking south along Torrey Pines Beach with the marine terraces clearly visible extending from the La Jolla coast to Mt. Soledad. |
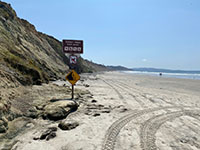
Fig 191. View looking south near northern boundary sign for Torrey Pines State Beach, located about a mile north of the bridge over the inlet. |
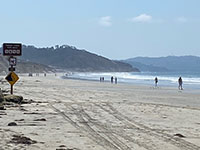
Fig 192. Zoom view looking south toward Torrey Pines Mesa near the sign marking the northern boundary of Torrey Pines State Beach. |
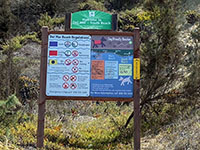
Fig. 193. Sign for Del Mar South Beach park boundary and rules sign located just north of the Torrey Pines State Beach boundary sign.
|
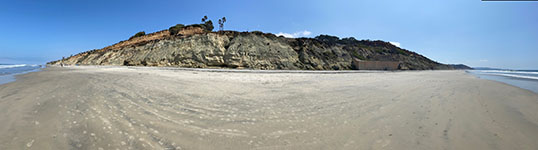
Fig 194. Panoramic view of the sea cliff on North Beach. The Delmar Formation of Eocene Age forms the pale-greenish layers in the sea lower sea cliff. The reddish-colored Bay Point Formation (above and to the left) is Late Pleistocene age associated with the Nestor marine terrace. North is to to left; south is to the right. |
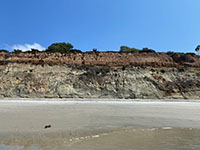
Fig 195. Greenish silty shale and sandstone channel deposit in the Delmar Formation with reddish Bay Point Formation
|
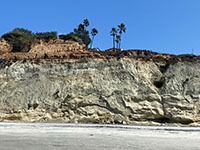
Fig 196. Greenish silty shale and a sandstone channel deposit in the Delmar Formation below reddish Bay Point Formation. |
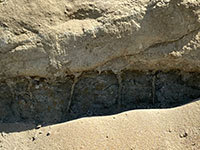
Fig. 197. Clastic dike filling in possible desiccation fissures beneath a younger conglomeration sandstone, (channel fill deposit).
|
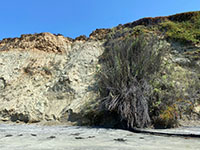
Fig 198. Fault with about 1 meter of vertical offset in the base of the Nestor Terrace deposits (brown on left) next to Del Mar Formation (green).
|
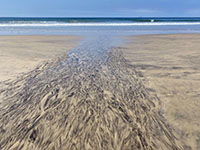
Fig. 199. Black sand sorted by a spring-water stream flowing down the beach. The black sand is mostly the mineral, magnetite. |
|
A25
Selected References and Resources
Abbott, P. L., 1999, The Rise and Fall of San Diego: 150 Million Years of History Recorded In Sedimentary Rocks: Sunbelt Publications, San Diego, CA, 231 p.
Beachapedia, 2018, State of the Beach/State Reports/CA/Beach Erosion: Beachapeida.org: http://www.beachapedia.org/State_of_the_Beach/State_Reports/CA/Beach_Erosion
Brown, J.E., [~1996], Geologic Formations of Western San Diego County: Geiconsultants.com, 7 p. [no longer available online].
California Geological Survey, 2020, Data Viewer (Geologic Map of California): https://maps.conservation.ca.gov/#dataviewer.
Givens, C.R., and Kennedy, M.P., 1979, Eocene molluscan stages and their correlation, San Diego area, California, IN Abbott, P.L., ed., Eocene depositional systems, San Diego, California; [field trip no. 18]: Geological Society of America Field Trip Guidebook, Joint Fall field trip of Society of Economic Paleontologists and Mineralogists, Pacific Section, and Geological Society of America, [92nd] annual meeting, San Diego, CA, November 8-9, 1979, no. 18, p. 81-95.
Google Earth, [2020]: https://www.google.com/earth/
Hanna, M.A., 1926, Geology of the La Jolla quadrangle, California: University of California Publications in Geological Sciences, v. 16, no. 7, p. 187-246, (incl. geologic map, scale 1:62,500).
Hertlein, L.G., and Grant, U.S., IV, 1939, Geology and oil possibilities of southwestern San Diego County: California Journal of Mines and Geology, v. 35, no. 1, p. 57-58.
Imbrie, J., Hays, J.D., Martinson, D.G., McIntyre, A., Mix, A.C., Morley, J.J., Pisias, N.G., Prell, W.L., Shackleton, N.J., 1984, The orbital theory of Pleistocene climate: Support from a revised chronology of the marine δ18O record, in Berger, A., Imbrie, J., Hays, J., Kukla, G., and Saltzman, B., eds., Milankovitch and climate: Understanding the response to astronomical forcing: Dordrecht, D. Reidel Publishing Company, p. 269-305.
Kennedy, G. L., 1973, A marine invertebrate faunule from the Lindavista Formation, San Diego, California: Transactions of the San Diego Society of Natural History, V. 17, no. 10, p. 119-127.
Kennedy, M.P. and Moore, G.W., 1971, Stratigraphic relations of Upper Cretaceous and Eocene formations, San Diego coastal area, California: American Association of Petroleum Geologists Bulletin, v. 55, no. 5, p. 709-722.
Kennedy, M.P. and Peterson, G.L., 1975,
Geology of the San Diego Metropolitan Area, California: California Division of Mines and Geology, Bulletin 200, 56 p. (with geologic maps).
Kennedy, M.P. and Tan, S.S., 2005, Geologic map of the San Diego 30' x 60' quadrangle, California: California Department of Conservation (see next reference).
Kennedy, M.P. and Tan, S.S., 2008, Geologic map of the San Diego 30' x 60' quadrangle, California: California Geological Survey Regional Geologic Map No. 3., Map Scale: 1:100,000, https://ngmdb.usgs.gov/Prodesc/proddesc_84173.htm; with pamphlet: https://ca.water.usgs.gov/projects/sandiego/data/gis/geology/kennedy2008/RGM3_San_Diego_2008_Pamphlet.pdf.
Kern, J.P., Stump, T.E., and Dowlen, R.J., 1971, An upper Pleistocene marine fauna from Mission Bay, San Diego. California. Trans. San Diego Society of Natural History Bulletin. 16, p. 329-338.
Kern, J. Philip and Rockwell, Thomas K.,1992, Chronology and Deformation of Quaternary Marine Shorelines, San Diego County California: In, Quaternary Coasts of the United States: Marine and Lacustrine Systems, SEPM (Society for Sedimentary Geology) Special Publication No. 48, p. 377-382.
Meldahl, K.H., 2015, Surf, Sand, and Stone: How Waves, Earthquakes, and Other Forces Shape the Southern California Coast. Oakland, CA: University of California Press, 222 p.
Meldahl, K., [2019], Geologic Evolution of San Diego County's Coasts and Lagoons: Mira Costa Community College (fieldtrip report):
http://h-m-herms.net/My%20Web/SELC-D/Geology/San_Diego_Coast%20(SEL%20docent%20version).pdf.
Moore, G.W., 1972, Offshore extension of the Rose Canyon Fault, San Diego, California, IN Geological Survey Research 1972: U.S. Geological Survey Professional Paper, 800-C, p. C113-C116.
Mueller, K., [2002?]. Origins of Coastal Uplift in San Diego and Orange Counties: Huge Blind Thrusts or Aseismic Rift Shoulder? USGS Research Grant, Final Report: Award Number 01HQGR0031: https://earthquake.usgs.gov/cfusion/external_grants/reports/01HQGR0031.pdf
Muhs, Daniel R., Webmiller, J.F., Simmons, K.R., and York, L.L., 2004,
Quaternary sea-level history of the United States: in The Quaternary Period in the United States, Gillespie, A.R. and Porter, S.C., Developments in Quaternary Science, v. 1, Elsevier Publ., p. 147-183.
NOAA, 2020, Climatological Datasets: National Oceanographic and Atmospheric Administration (NOAA), National Centers for Environmental information: https://www.ncdc.noaa.gov/data-access/paleoclimatology-data/datasets.
San Diego History Center, 2020, Timeline of San Diego History: https://sandiegohistory.org/archives/biographysubject/timeline/
Schulz, Marjorie, Lawrence, C, Muhs, D, Prentice, C., and Flanagan, S., 2018, Landscapes from the Waves—Marine Terraces of California: USGS Fact Sheet: https://pubs.usgs.gov/fs/2018/3002/fs20183002.pdf .
Spratt, R.M. and Lislecki, L.E., 2016, A Late Pleistocene sea level stack: Climate of the Past, v. 12, p. 1079-1092.
Stamm, N., 2000, U.S. Geological Survey, Geolex—Significant Publications:
https://ngmdb.usgs.gov/Geolex/search.
Stright, L. Stweart, J. Camion, K. and Graham, S., 2014, Geologic and seismic modeling of a coarse-grained deep-water channel reservoir analog (Blacks' Beach, Lajolla, California: AAPG Bulletin 98 (4), p. 695-728.
Tan, S.S. and Kennedy, M.P., 1996, Geologic Maps of the Northwestern Part of San Diego County, California, Open File Report 96-02, California Division of Mines and Geology.
Torrey Pines Association website [2020]: https://torreypines.org/. This website has a wealth of information not presented here, particularly about the wildlife biology, ecology, geology, and history and management of the Torrey Pines State Nature Preserve.
U.S. Board on Geographical Names, U.S. Geological Survey, [2020], Domestic Names [This database provides names, coordinates, and elevations of named places]: https://www.usgs.gov/core-science-systems/ngp/board-on-geographic-names/domestic-names.
|
https://gotbooks.miracosta.edu/fieldtrips/torrey_pines/index.html |
9/24/2021 |
|