Chapter 6 - Marine Sediments |
6.1
This chapter is about the origin and distribution of sedimentary deposits (sediments and sedimentary rocks) with a focus on marine sediments.
The word “sedimentary” refers to materials consisting of sediments or formed by deposition; the word sedimentary also applies to both the processes and the products of deposition (Figure 6-1).
Sediment: Solid material that has settled from a state of suspension. Sediments are transported and deposited by water (rivers, lakes, and oceans), ice (glaciers), and wind.
Sedimentary rock is rock that has formed through the deposition and consolidation and solidification of sediment. Sedimentary rocks are often deposited in layers, and frequently contain fossils. Studies of sedimentary deposits can help tell the geologic history of an area. |
Click on images for a larger view throughout this website. |
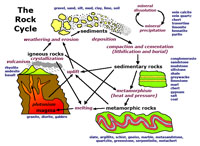 |
Fig. 6-1. Sediments, sedimentary rocks and sedimentary processes are part of the Rock Cycle. |
6.2
Classification of Sediments and Sedimentary Rocks
Sediments (and sedimentary rocks) are classified in by origin of source material and by grain size.
There are 4 sources of sediments (and sedimentary rocks):
a) Cosmogenous: material that falls to the Earth surface from outer space.
b) Hydrogenous: material precipitated directly seawater.
c) Lithogenous: material derived from erosion of other rocks, typically from continental sources.
d) Biogenous: material formed from the accumulation of remains of living organism.
|
6.3
Cosmogenous Sediments
Cosmogenous sediments originated from outer space. Scientists have used satellites to estimate how much material enters the earth's atmosphere. Current estimates from satellite data suggesting about 100 to 300 tons (mostly cosmic dust) hits earth each day. This is just a tiny fraction of the sediments generated on earth each day. However, early in the history of our Solar System, Earth and other planets, moons, comets and asteroids formed from the gravitational accumulation of extraterrestrial material, but by 4.5 million years ago, most of this cosmogenous accumulation had significantly diminished. However, cosmogenous materials including iron-nickel and stony meteorites can be found. Although a relatively insignificant source of sediment, meteor fireballs disintegrating in the atmosphere contribute dust that can accumulate measurable amounts in parts of some ocean basins.
Extraterrestrial impacts have changed life on Earth repeatedly, including the mass extinction at the end of the Mesozoic Era associated with the extinction of dinosaurs and many other forms of life on land and in the oceans. Tektites are silica glass generated by extraterrestrial impacts: asteroids exploding on the surface and molten material is ejected into the atmosphere where it condenses into a glass-like material. |
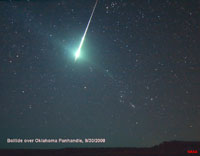 |
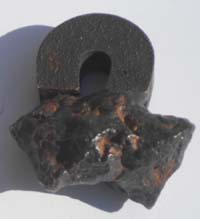 |
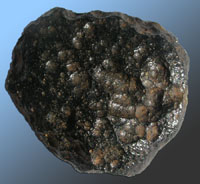 |
Fig. 6-2. A meteor fireball (a bolide) disintegrates in the night sky over Oklahoma. |
Fig. 6-3. Iron-nickel meteorite from the Diablo Canyon area, AZ (see below) |
Fig. 6-4. A tektite is a ball of glass-like material ejected by an asteroid impact. |
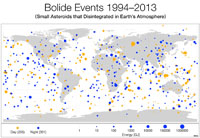 |
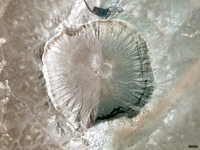 |
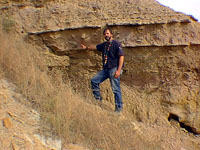 |
Fig. 6-5. Known locations of bolide events (1994 to 2013). Bolides are meteor fireballs that explode when entering the atmosphere. Few reach the ground or oceans. |
Fig. 6-6. Meteor Crater (Diablo Canyon site) near Flagstaff Arizona is a 50,000 year-old asteroid impact site about a mile in diameter and 550 feet deep. |
Fig. 6-7. The Cretaceous-Tertiary extinction event is preserved in sediments in many locations around the world. This one is in South Dakota. |
6.4
Hydrogenous Sediments
Hydrogenous sediments are sediments directly precipitated from water. Examples include rocks called evaporites formed by the evaporation of salt bearing water (seawater or briny freshwater).
Evaporites (Salts)
An evaporite is a rock composed of salt minerals left behind by the evaporation of salty water. Examples include minerals halite [salt] (NaCl) and gypsum (CaSO4 • x H2O).
rock salt—a rock dominantly composed of sodium chloride (NaCl - the mineral halite; Figure 6-8). Rock salt is an evaporite formed in restricted basins with an inflow of seawater located in an arid environmental setting.
gypsum—a mineral composed of hydrous calcium sulfate (CaSO4-2H2O); an evaporite mineral used in the manufacture of plaster. Gypsum is deposited by concentrated seawater and by evaporation of freshwater in arid regions. Crystals of gypsum are common in soils in arid regions. If gypsum looses its water content, it is called anhydrite (Figure 6-9).
Salts are precipitated when sea water (or briny lake water) is concentrated by evaporation. Shorelines along the oceans in hot arid regions of the world are places where salt, gypsum and anhydrite are being deposited today. Places where salts (evaporites) are actively accumulation include around the Red Sea and Persian Gulf. Salt deposits are also forming in isolated, internally drained lake basins around the world including the Great Salt Lake in Utah and the Dead Sea.
Iron-manganese nodules
Iron-manganese nodules form on the ocean bed (mostly in the deep Pacific) from the slow precipitation of metal oxides in the absence of other kinds of sediments. It may take many millions of years for an individual manganese nodule to grow on the deep seafloor. Deposits of them cover the seafloor only in regions located very far away from lithogenous sediment sources.
|
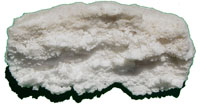 |
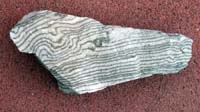 |
Fig. 6-8. Rock salt (halite) |
Fig. 6-9. Anhydrite gypsum. |
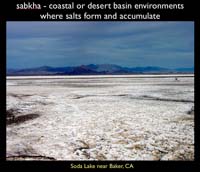 |
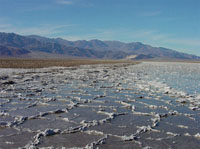 |
Fig. 6-10. A sabkha is a desert coastal environment is where salts, including halite and gypsum, are commonly deposited. |
Fig. 6-11. Polygons of salt crystals (halite) being deposited on a salt pan on a dry lake bed in Death Valley National Park, California |
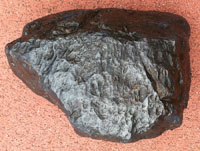 |
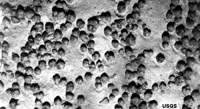 |
Fig. 6-12. An iron-manganese nodule forms very, very slowly from direct precipitation on the seafloor. |
Figure 6-13. Iron-manganese nodules on the deep sea floor near the Puerto Rico Trench. |
6.5
Lithogenous Sediments
Lithogenous sediments form through the processes of weathering and erosion of materials exposed on land and along coastlines. Lithogenous sediments consist of solid fragments of inorganic or organic material that come from the weathering of rock and soil erosion, and are carried and deposited by wind, water, or ice. Lithogenous sediments are also commonly called "terrigenous sediments" because they are derived dominantly from terrigenous (land) sources. They are also called "clastic sediments" because they are made up of rock fragments derived from other rocks—a "clast" is a Greek word for a rock fragment.
Lithogenous sediments are:
• Mostly small pieces of broken rock transported to ocean from the land (wind, rivers, glaciers, coastal erosion, turbidity currents etc.)
• Generally form deposits rapidly (such as sand on a beach or a river delta)
• Can form in high energy environments and have coarse grain sizes (coarse sand, gravel, cobbles, and boulders).
• Beach sand is mostly composed of the quartz (SiO2), a mineral which very resistant to weathering.
• Most lithogenous sediments eventually are deposited along the margins of ocean basins.
• Some is deposited into the deep ocean by currents and underwater landslides near continents, and far offshore, lithogenous sediment of fine silt and clays, some as desert dust, forest-fire ash, or volcanic ash blown in by the wind. |
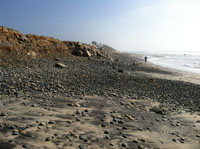 |
Fig. 6-14. Sand and gravel on SoCal beaches are typical lithogenous sediments. This view is of South Carlsbad State Beach, California. |
|
6.6
Biogenous Sediments
Biogenous sediments are composed of the remains of living organisms, including microscopic phytoplankton (plants) and microscopic zooplankton (animals), terrestrial and aquatic plants, shells of invertebrates, and vertebrate material (teeth, bone), and associated organic residues. Coal, oil, and gas are derived from biogenous sediments. Biogenous sediments accumulate to form massive deposits associated with modern and ancient carbonate "reef systems" (such as the Australian Barrier Reef, South Florida, Keys, and the Bahamas, the Yucatan and reefs throughout the Caribbean Sea, and great reefs and atolls in thou gout the South Pacific, Indian Ocean, and many other locations.
(See more on Biogenous Sediments below.) |
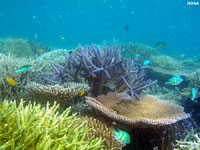 |
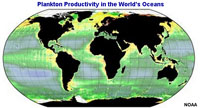 |
Fig. 6-15. Biological activity creates large volumes of sediment in some ocean regions. |
Fig. 6-16. Oceanic plankton constitute the largest reservoir of biomass in the world's oceans |
6.7
Neritic and Pelagic Sediments
The term neritic is used to described the shallow part of the ocean near a coast and overlying the continental shelf.
Neritic sediments are generally shallow water deposits formed close to land. They are dominated by lithogenous sources and are typically deposited quickly. Neritic sediments cover about ¼ of sea floor and are near landmasses.
The term pelagic means "of or relating to the open sea" particularly the upper layers of the ocean away from shore.
Pelagic sediments are generally deep-water deposits mostly oozes (see below) and windblown clays. They are typically finer-grained sediments that are deposited slowly. Because they are deposited far beyond the continental margins they are typically less lithogenous and more biogenous depending on biologic productivity. Pelagic sediments cover about ¾ of seafloor and are mostly in deep water.
The distribution of neritic or pelagic sediments is controlled by proximity to sources of lithogenous sediments (i.e.: landmasses) and the productivity of microscopic marine organisms. |
6.8
Volume and Distribution of Marine Sediments
Of the 4 types of sediments, lithogenous and biogenous sediments are the most abundant on Earth today. Lithogenous sediment dominate the regions adjacent to continental landmasses (continental margins). The lithogenous sediment accumulations along continental margins can be many miles thick, especially where rivers have dumped large quantities of sediments for long periods of geologic time. Biogenous sediments accumulations can also be massive, particularly in locations where warn, shallow seas allow massive reef tracts to persist for long periods of time, such as with the Australian Great Barrier reef. Planktonic remains blanket the seafloor in large regions of the world's oceans. In contrast, cosmogenous and hydrogenous sediments are generally insignificant in comparison, but have important scientific and economic significance where they occur.
Sedimentary rocks are exposed throughout the world's continents, covering about half of the exposed land on the earth surface. This sedimentary cover blanketing continental areas was originally deposited mostly in coastal environments, in shallow seas flooding shallow continental basins, on continental shelves and in ocean basins along the margins of continents. Most of these sedimentary rocks that blanket much of the continents formed in the last several hundred million years. Even more massive quantities of sediments occur along continental margins in ocean basins. In many places around the world the thickness of sediments eroded from continental landmasses and volcanic chains and deposited in the adjacent ocean basin can be many miles thick! Sediments are thinnest or nonexistent on new ocean crust forming along mid-ocean ridges.
Most lithogenous sediments are on or near a landmass
• Coarser sediments accumulate closer to shore,
• Finer sediments are winnowed by waves and currents and are transported farther from shore to quieter water settings where they can settle out. |
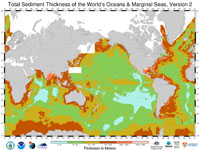 |
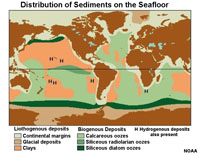 |
Fig. 6-17. Thickness of sedimentary deposits along continental margins. |
Fig. 6-18. Distribution of sediments on the seafloor by type. |
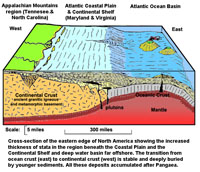 |
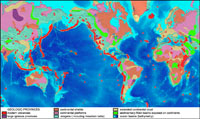 |
Fig. 6-19. Continental margins are places where large quantities of lithogenous and biogenous sediments accumulate. They are thinnest or missing on new ocean crust forming on mid-ocean ridges. |
Fig. 6-20. Map of geologic provinces of the world. Sediments and sedimentary rocks not only cover much of the world's seabed but also cover large regions of the continents that were once under water. |
6.9
"High-Energy" and "Low-Energy" Depositional Environments |
Flowing water is the dominant natural force causing erosion and deposition on Earth. The faster the water moves, the higher the energy in a physical setting. As flowing water increases in speed, the more it may become turbulent, increasing its ability to lift and move particles. Fast moving water can carry materials of different sizes ranging from boulders and gravel to finer materials (sand, silt, and clays). Flowing water also sorts sediments by size and density. High-energy environments include river channels, beach and shallow offshore environments with high wave action, and wave-battered coral reefs (Figures 6-21 to 6-22). Fast flowing water from waves and currents may let larger materials settle and be deposited while finer materials are carried away and deposited in quieter water settings or what are considered low-energy environments. (Figures 6-23 to 6-24). Low-energy environments on land include most lakes and swamps, and low-energy conditions exist in protected bays and lagoons, and in deeper-water setting in ocean in locations not significantly impacted by wave and strong current action.
Different sedimentary environments have different energy characteristics that may change from time to time. The forces of energy in a stream will increase as the volume of water increases, such as during flood. For most of a year, a stream will may be a calm environment, that changes during a flood, or during a flood season. The same is true of beach and offshore bar environments. As wave energy increases, the greater the amount of energy translates into shoreline erosion and the moving of sediments to quieter and deeper offshore settings. Wave action separates sand from courser and finer fractions, building up or eroding beaches with changing conditions. A beach or offshore region can remain basically calm, relatively low energy for years until a hurricane comes along, and the setting becomes "high energy." One big storm event can move more sediments in a few days that might have moved for decades or even centuries. For example, Hurricane Camille did this to the coast of Alabama and Mississippi in 1969.
Deep-water environments far from shore tend to be low energy environments. However, in regions along continental margins quiet conditions can be suddenly disrupted by the rapid influx of sediments caused by massive underwater landslides or the effects of major storms on the nearby continental shelves.
|
High-Energy Depositional Environments
Coarse-grain sediments dominate. Weather (climate), currents, and wave energy are variable factors in "high-energy" environmental settings. Sediments are constantly being deposited or eroded in these settings. |
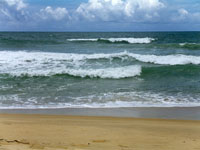 |
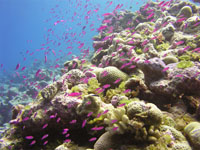 |
Fig. 6-21. Beaches |
Fig. 6-22. Coral reefs |
Low-Energy Depositional Environments
Fine grained sediments dominate. Slow-moving currents prevent coarse-grained sediment from migrating into in low-energy settings. Fine materials can be carried long distances before they can settle out in the absence of waves and currents.
|
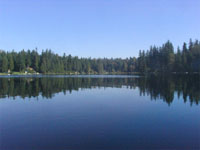 |
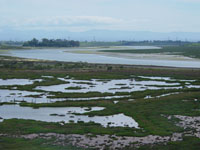 |
Fig. 6-23. Lake (lacustrine) and swamp environments. |
Fig. 6-24. Tidewater marsh and estuary/lagoon settings. |
Sedimentary deposits preserve aspects of the energy levels of the locations in which they were deposited.
In general, the particle size of sediments is larger in sedimentary deposit deposited in high-energy environments. Fine-grained sediments tend to erode in high energy environments, and tend to be deposited in low energy environments. In addition, the higher energy environments tend to have higher dissolved oxygen and nutrient concentrations, which influences the kind of organisms that live in such environments. |
6.10
Sources of Lithogenous Sediments: Continental Weathering and Erosion
Rocks on or near the surface are exposed to physical and chemical interactions with air and water. The breakdown of earth materials due to exposure is called weathering. Weathering produces sediments; erosion moves sediments.
Lithogenous sediments are solid fragments of inorganic or organic material that come from the weathering of rock. On land and under water sediments are subjected to gravitation forces pulling them downslope. Erosion is the mechanical and chemical processes of weathering, wearing or grinding away materials on a landscape by the action of wind, flowing water, or glacial ice. Deposition is the process of sediments settling and accumulating from a moving fluid (wind, water, or ice). Once sediments have accumulated in a stable setting they can gradually undergo compaction and cementation to form sedimentary rocks.
Sediments can be eroded, transported, and deposited, often over and over again. Most sedimentary deposits preserve evidence about how, when, where, and why they were deposited! |
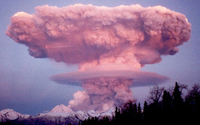 |
Fig. 6-25. Volcanic eruptions can produce large volumes of ash and other debris that can be eroded, transported, and deposited as marine sediments. |
6.11
Weathering
Weathering is the gradual destruction of rock under surface conditions. Weathering may involve physical processes (called mechanical weathering) or chemical activity (called chemical weathering). Biological activity can also result in weathering that can be construed as mechanical, chemical, or both.
Weathering processes can begin long before rocks are exposed at the surface. This is true in most places on the earth surface where rocky outcrops (bedrock) is not exposed. In addition, weathering and erosion can take place simultaneously, perhaps most obviously in settings like rivers in flood, or waves crashing on a beach.
Mechanical Weathering
Mechanical weathering involves all processes that collectively break rocks into smaller pieces. Mechanical weathering includes all forms of mass wasting—a general name for processes by which soil and rock move downslope under the force of gravity. Mass wasting, a form of mechanical weathering, includes sudden events such as rock falls, landslides, slumps, and avalanches. These processes break "big pieces of rocks into smaller pieces."
Mechanical weathering can involve erosional grinding as fast-moving flood waters moves boulders and sediments down stream valleys and where wave action batters rocks into sand along a shoreline. Rocks are shattered by earthquakes and volcanic explosions, the expand and split when erosion unloads overburden on compressed rocks that were previously deeply buried. Rocks will split when water freezes and expands in cracks. Rocks exposed on the surface are subject to expansion and contraction caused by daily heating and cooling (particularly effective in arid environments). Mechanical weathering is also caused by organic activity—the breakdown and movement of rock and soil caused by expanding tree roots, burrowing, feeding activity, etc.
The mechanical breakdown of rocks increases the surface area (per unit area) increasing the available surface area where chemical weathering can take place (Figure 6-21). |
|
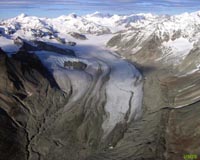 |
Fig. 6-26. Mechanical weathering is any process that makes "big pieces into smaller fragments." |
Fig. 6-27. Glaciers (moving ice) scours bedrock and produce and carry away large quantities of sediment. |
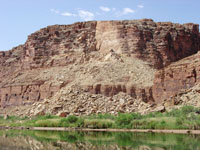 |
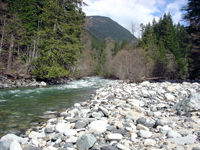 |
Fig. 6-28. Gravity drives mass wasting. In this case, a rock fall, breaks big pieces into fragments. |
Fig. 6-29. Flood waters can move all sizes of sediments, when the water slows down, sediments are deposited. |
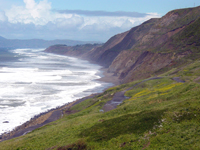 |
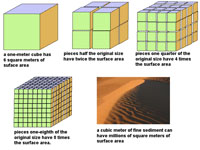 |
Fig. 6-30. Coastal Erosion by wave, tides, and current is a major source of lithogenous sediments. This view is of the Thornton Beach coastal landslide area near San Francisco, California). |
Fig. 6-31. The mechanical breakdown of rocks increases surface area (per unit volume). Increased surface area increases the space for chemical weathering processes to take place. |
6.12
Chemical Weathering
Chemical weathering involves the breakdown (decomposition, decay, and dissolution) of rock by chemical means. Dissolution is the action or process of dissolving or being dissolved, moving soluble components of materials into solution. Leaching is the process of dissolving and removing the soluble constituents of soil or rock near the land's surface. Water flowing under the influence of gravity carries dissolved materials away, ultimately adding to the saltiness of the oceans or they are deposited as salts, such a such as in an inland desert dry lake basin.
In most surface and near surface settings, mechanical and chemical weathering are taking place simultaneously.
Weathering and erosion are continuous processes in the surface environment, enhanced by the presence of water. Water is commonly called the universal solvent because so many compounds can be dissolved in it.
The journey of sediments can take a long time! The migration of sediments from upland regions to the ocean basins can take a very long time. Sediments can eroded and re-deposited many times along the journey.
|
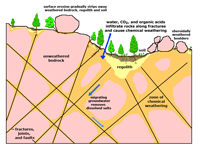 |
Fig. 6-32. Weathering involves many processes occurring at or near the surface environment. Fractures allow water and air to penetrate into the bedrock allowing chemical weathering processes to take place. |
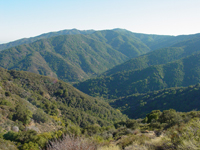 |
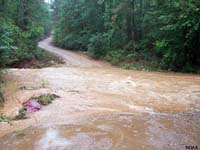 |
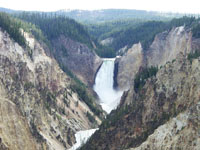 |
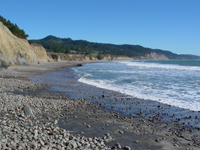 |
Fig. 6-33. Weathering is most intense where water is present (such as in upland areas that receive greater precipitation). This view shows Loma Prieta Peak near San Jose, CA. |
Fig. 6-34. Flood waters carry sediments: large particles (boulders, gravel, and sand) roll and bounce along on the stream bed; finer materials (fine sand, silt, and clay) can be carried in suspension. |
Fig. 6-35. Flowing water transports, grinds fragments, and erodes landscapes. Stream and river erosion are dominant forces changing mountainous landscapes. They contribute most of the sediments that build beaches and shoreline deposits. |
Fig. 6-36. Wave action along shorelines grinds rocks into fragments. Storm-driven currents in ocean and lake settings and wave action along coastlines can move tremendous amounts of sediments. (Año Nuevo State Beach, California) |
|
6.13
Fate of soluble components of rocks: formation of seawater
As rocks weather and erode, they loose their soluble elemental components, they dissolve in groundwater and surface runoff and are carried away, eventually reaching the ocean. The high level of salt in seawater comes from\ the weathering and erosion of rocks on the surface or the seafloor. Salts dissolved in water flowing off of the continents or water flowing through sediments or rocks underground. Evaporation concentrates salt in seawater. Salt concentrations higher than seawater occur isolated lake basins in arid regions, such as in North America's Great Basin region. Salts end up being concentrated as salts on dry lake beds—or brine in basins such as Mono Lake (CA) or the Great Salt Lake (Utah). Over billions of years, rivers and streams, and groundwater flowing into the oceans have contributed to the saltiness of seawater. Salt in seawater is concentrated by the evaporation of water back into the atmosphere. (See Why is the ocean salty?) |
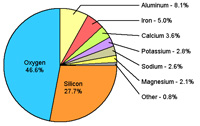 |
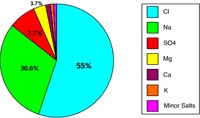 |
Fig. 6-37. Composition of crustal rocks, some elements are more soluble and others. |
Fig. 6-38. Elemental components of salts dissolved in seawater. |
6.14
Sediments Classification Based On Grain Size |
Sediments are solid fragments of inorganic or organic material that come from the weathering of rock and soil erosion, and are carried and deposited by wind, water, or ice. They range in size from large blocks to microscopic particles. Figure 6-39 shows the technical definition of sediment particles. However, general usage is as follows ranging from largest to smallest: boulders, cobbles, gravel, sand, silt, and clays.
Sediments form from the disintegration of rocks. They are transported, mostly by water, and in the process the fragments are abraded, with sharp edges worn down and the overall shape of particles increasing in roundness. Sediments derived from erosion on land are mostly lithogenous sediments.
Clastic sediments and sedimentary rocks
The word clastic is also commonly used to describe sediments or sedimentary rocks composed of fragments (or detritus) derived from older rocks. The word clast means rock fragment; the word is derived from the Greek word klastos which means broken. Gravel, sand, and silt are examples of clastic sediments. Lithogenous sediments (described above) are mostly clastic sediments. A classification of clastic sediments and sedimentary rocks is illustrated in Figure 6-39 and with details discussed below. |
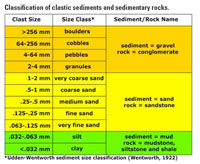
Fig. 6-39. Classification of sediments by size of clasts (rock fragments) |
6.15
How do sediments become sedimentary rocks?
Sediments can become lithified into sedimentary rocks once they've been deposited in a stable setting where burial, compaction, and cementation can take place. The processes, collectively called lithification (or diagenesis) typically takes place slowly over time but rates depend on many factors including the chemistry of the sediments and groundwater passing through the sediment, and how quickly or deeply burial takes place. Deposits of unconsolidated sediments typically have high porosity—pores are open spaces between grains filled with gas or fluids (water or in some cases, petroleum). Compaction is the process of gravitation consolidation of sediments, decreasing the volume of pore space between particles of sediment and increasing hardness. Cementation involves processes that harden sediments through the precipitation of minerals in pore spaces between grains of rock and mineral fragments, binding them together (Figure 6-40). Common minerals that form cement include quartz, calcite, limonite, hematite, and clays. The cementing minerals are slowly deposited between grains by groundwater.
|
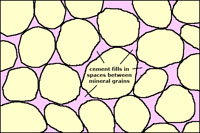 |
Fig. 6-40. Cementing minerals fill in spaces between sediment grains as they turn to stone. |
6.16
Clastic Sedimentary Rocks
Rocks composed of grains of mineral and rock fragments derived from erosion of other rocks. Three general groups are coarse-grained, sand-size grained, and fine-grained ("mudrocks").
Coarse-grained sediments and sedimentary rocks
Gravel is rock particles that have been moved by moving water. Gravel usually consists of a mix of the more durable and most abundant rock types in the sediment source areas (Figure 6-41). Gravel deposits typically occur along stream valleys close to mountainous source areas and along rocky coastlines with high wave action.
Conglomerate is a sedimentary rock composed of cemented gravel. It consists of rounded to sub-angular fragments (larger than 2 mm in diameter) set in a fine-grained matrix of sand or silt, and commonly cemented by calcium carbonate, iron oxide, silica, or hardened clay; the consolidated equivalent to gravel (Figures 6-42). The composition of grave reflects the rocks the general composition in the area where it comes from.
Sand-size-grained sediments and sedimentary rocks
Sand goes through degrees of refinement at it moves away from source areas. Sand deposits near mountain ranges may be enriched in feldspars. Volcanic regions may produce sand enriched in dark minerals. "Mature" sand that has traveled long distances in streams, blown by wind, or worked and reworked by waves will be enriched in quartz and individual grains will be very well rounded and well sorted (see below). Large sand deposit accumulate along stream valleys, on beaches, barrier islands, and offshore bars, and in dune fields in coastal areas and in desert environments.
Sandstone is a sedimentary rock formed by the consolidation and compaction of sand and held together by a natural cement, such as silica, calcite, and iron-oxide minerals (Figure 6-44). Most sandstone is dominated by the minerals quartz.
Fine-grained sediments and sedimentary rocks
("Mudrocks")
Mud is a general term lumping together sediments consisting of a mix of clay, silt, and may contain sand. Mud is usually an unsorted mix of fine grain materials. Mud accumulates in quiet water settings separated from where coarser materials have settled out elsewhere (Figure 6-46). Most soil is mud. Mud-rich accumulations are common in river delta regions, swampy coastal regions, tidal flats, and in lake and deep water settings.
Mudstone is a fine-grained sedimentary rock formed from the compaction and cementation (lithification) of muddy sediments rich in silt (but may include percentages of fine sand and clay).
Shale is a soft, finely stratified sedimentary rock that formed from consolidated mud rich in clay minerals and can be split easily into fragile plates, such as along bedding plains. Shale forms from the compaction of sediment dominated by clays.
Clays are composed of any microscopic mineral particles. Most dust is clay sized particles. However, there are several types of clay minerals. Clay minerals are any of various hydrated aluminum silicates that have a fine crystalline structure and are components of clay (sediment). Clay minerals form from the weathering of feldspars and other silicate minerals and are the dominant sediment found on earth.
Graywacke (or graywacke or grauwacke, a German word signifying a gray, earthy rock) is a variety of sandstone or mudrock generally characterized by its dark color and poorly sorted angular grains including a mix of quartz, feldspar, dark mafic minerals, and tiny rock fragments cemented in a compact, clay-fine matrix. Generally, graywacke is a featureless dirty-looking, dark brown or gray sandstone or silty mudstone. Graywacke is common in active continental margin regions such as along coastal California (Figures 6-49 and 6-50). |
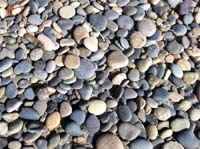 |
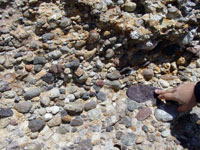 |
Fig. 6-41. Wave action creates well rounded and sorted gravel. |
Fig. 6-42. Conglomerate formed from an ancient gravel deposit. |
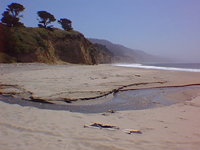 |
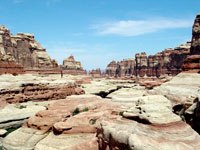 |
Fig. 6-43. Sand is winnowed (sorted) and accumulates on a beach by wave action. |
Fig. 6-44. Sandstone outcrops exposed in Utah's Canyonlands National Park |
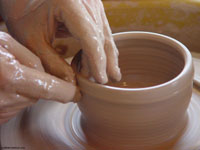 |
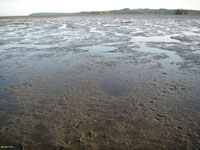 |
Fig. 6-45. Certain kinds of Clays are used to make ceramic pottery. Clay is made up of clay minerals. |
Fig. 6-46. Mud accumulates in quiet-water environments as illustrated with these tidal flats.
|
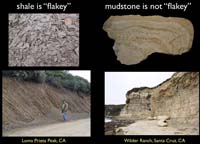 |
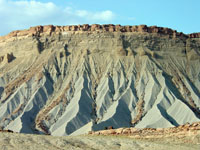 |
Fig. 6-47. Comparison of shale and mudstone. Shale tends to be flaky and splits into thin layers. Mudstone tends to form more massive layers. |
Fig. 6-48. Shale (blue gray) and mudstone (brown) outcrops in Utah's Capitol Reef National Park. Marine shales tend to be shades of blue, green, and gray.
|
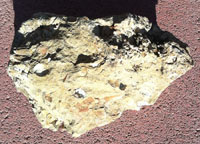 |
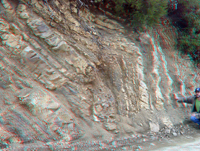 |
Fig. 6-49. Graywacke is a poorly sorted mix of sediments common in marine deposits along active continental margins. |
Fig. 6-50. Graywacke is perhaps the most abundant sedimentary rock exposed mountainsides throughout coastal California. |
6.17
Unique characteristics of lithogenous deposits
Sediments preserve other characteristics that may tell information about the environment where they occur. Sediment particle shapes (rounding), degree of sorting, and bedding characteristics are typically unique to different geologic settings.
Rounding of sediment grains
When particles are moved by running water they become rounded ("roundness" is illustrated in Figure 6-51). The corners hit
first and are worn down. The sharp edges are also pounded. The particles may become round
boulders or pebbles. Bits of sand move with them. As the water slows the largest particles drop
out first, making
deposits of round
boulders and pebbles
called conglomerate. The smaller
particles are swept away
downstream (unless they
are trapped between or
beneath the large
particles). |
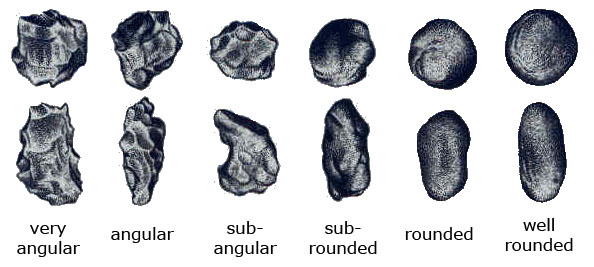 |
Fig. 6-51. "Roundness" of sediment grains: The farther a particle is moved, the more rounded and
spherical it should become. Angular particles tend to be deposited close to
their source, they become more rounded the farther they travel downstream. Grains of beach sand are typically well rounded. Dune sand is typically even more rounded and better sorted (Image from Powers, 1959). |
6.18
Sorting
The ability of running water to move sediments also sorts particles by size and to a lesser degree by
shape. This is called sorting (illustrated in Figure 6-52). Sediments exposed to longer transport or exposure to currents and waves tend to be more sorted by shape and size.
The amount of sorting depending on the energy conditions and
amount of time at which the stream currents or ocean waves works on the particles. For instance, particles of the same
mineral that are more rounded and more sorted have traveled farther.
|
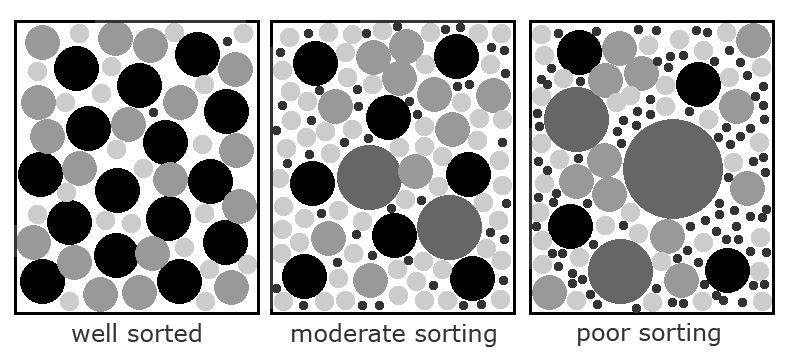 |
Fig. 6-52. Sorting of sedimentary particles. Beach sands the to be very well sorted. River sands tend to be moderately sorted. Deep ocean turbidity current sediments tend to be very poorly sorted.
|
As transportation distance increases, sediment becomes more "mature" and:
• Clay content decreases (clays are carried away and deposited in other quiet water settings)
• Sorting increases (gravel and sand gets concentrated)
• Non-quartz minerals decrease (quartz is both an abundant and is harder than other common minerals)
• Grains become more rounded (sharp edge break off easier) |
The sediments sorting,
roundness, and sphericity could act as a clue to following either modern or ancient alluvial
rocks to their ultimate source (such as for finding gold and diamonds). For example, very well sorted and rounded materials may suggest a source
from an older sedimentary rock rather than from freshly exposed igneous rocks. Sand from rivers and stream are very different from sands associated with beach and sand-dune deposits (see Figures 6-53 to 6-56). |
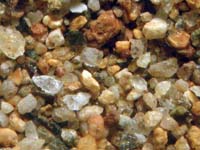 |
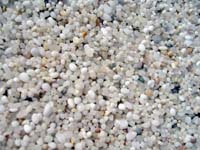 |
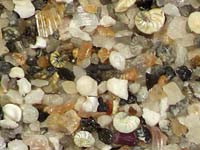 |
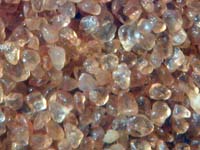 |
Fig. 6-53. Sand from a mountain stream may be rich in poorly sorted and angular grains of feldspars, quartz, and other minerals. |
Fig. 6-54. Beach sand is enriched in well rounded and consist mostly of well-sorted quartz grains. Fine materials are winnowed out. |
Fig. 6-55. Beach sand in many tropical settings may be enriched in shell material, including microfossils (such as shells of foraminifera). |
Fig. 6-56. Wind-blown dune sand is typically very well sorted and very well rounded, polished to frosted grains of mostly quartz. |
|
6.19
Sedimentary Processes and Sedimentary Structures
Lamination and bedding
Sediments are deposited in layers ranging from paper-thin sheets to massive beds tens to hundreds of feet thick! A laminae (or lamination) is a layer of sediment or sedimentary rock layer only a small fraction of an inch (less than a centimeter) in thickness (see Figure 6-57). Thin lamination is typically associated with fine-grained sediments deposited in quiet or slack-water environments, such as in a lake basin or offshore below the influence of waves and strong currents. Bedding is the smallest division of a sedimentary rock formation or stratigraphic rock series marked by well-defined divisional planes (bedding planes) separating it from layers above and below (see Figure 6-58).
|
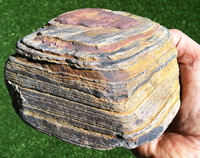 |
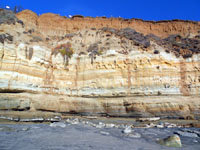 |
Fig. 6-57. Lamination in shale. Each laminae may be an annual cycle of deposition or a seasonal storm flood event (scale is in mm to cm). |
Fig. 6-58. Bedding is layers of sediment deposited in an environmental setting on a scale of hundreds to many thousands of years. |
6.20
Sedimentary structures preserved in bedding
Sedimentary deposits (including sediments and sedimentary rocks) commonly preserve evidence of how they were deposited. Anyone who has been to the beach or a sand dune area have seen ripple marks created by the movement of sand under the influence of wind or water. Listed below are examples of sedimentary structures preserved in bedding of ancient sedimentary rocks. The processes that created them are the same that can be observed occurring today.
ripple marks—a series of small ridges produced in sand by water currents or by wind (Figure 6-59).
cross bedding—inclined sedimentary structures in a horizontal unit of rock. These tilted structures are deposits from bedforms such as ripples and dunes, and they indicate that the depositional environment contained a flowing fluid (typically, water or wind) (Figure 6-60 and 6-61).
desiccation cracks—mudcracks; irregular fracture formed by shrinkage of clay, silt, or mud under the drying effects of atmospheric conditions at the surface (Figure 6-58).
graded bedding—bed is one characterized by a systematic change in grain or clast size from the base of the bed to the top. Large fragments tend to settle out fastest from a slowing turbulent flow.
biological structures—many kinds of organisms burrow or bore into sediments creating holes for feeding or for shelter (or both). Most marine sedimentary beds preserve bioturbation features - bioturbation means "churning of the sediments" as organisms, typically worms, shrimp, and other invertebrates work through the sediments to eat decaying organic mater (or other organisms feeding there). They also use the burrows as shelter or nesting site. Very often the traces are preserved as structures in the sediment. Trackways, burrows, or resting sites are also common structures preserved in marine sediments.
Turbidity Currents and Development of Submarine Canyons and Fans
A turbidity flows is a turbid, dense current of sediments in suspension moving along downslope and along the bottom of a ocean or lake. In the ocean, turbidity currents can be massive episodic events. They typically form and flow down through a submarine canyon (carved by previous turbidity flows) and accumulate near the base of the continental slope on deep-sea fans. Turbidity flows produces deposits showing graded bedding (Figure 6-63 and 6-64). Slowing turbid currents drop their coarser fractions first (gravel and sand) and the finer silt and clay fractions settle out last. |
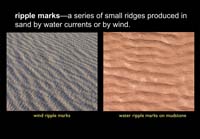 |
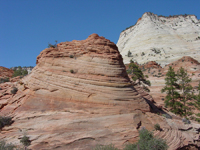 |
Fig. 6-59. Ripple marks on sand dune sand in water deposits form from current flow (air or water) |
Fig. 6-60. Cross bedding in ancient sand dune deposits
Zion National Park, Utah |
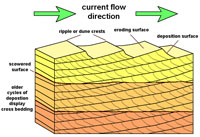 |
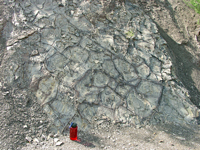 |
Fig. 6-61. Formation of cross bedding caused by the migration of ripples or dunes |
Fig. 6-62. Desiccation mud cracks in Precambrian rocks,
Grand Canyon, Arizona |
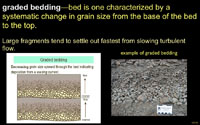
Fig. 6-63. Appearance and example of graded bedding in sedimentary deposits. Graded beds will "fine-upward" as currents slow down. They may "coarsen upward" if the energy of the depositing flow (current) increases. |
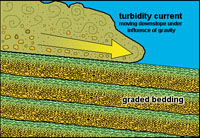
Fig. 6-64. Turbidity currents flow down slope under water under the influence of gravity. At peak flow, turbidity currents will scour the seabed, but as flow slows and stops, coarse sediments are deposited first, and finer material last. |
6.21
Deep Sea Fan and Turbidite Deposits
A deep-sea fan is a fan- or delta-shaped sedimentary deposit found along the base of the continental slopes, commonly at the mouth of submarine canyons. Deep sea fans form from sediments carried by turbidity flows (density currents) that pour into the deep ocean basin from the continental shelf and slope regions and then gradually settle to form graded beds of sediment on the sea floor. Deep-sea fans can extend for many tens to hundreds of miles away from the base of the continental slope and an coalesce into a broad, gently sloping region called a continental rise.
Graywacke is a fine-to-coarse-grained sedimentary rock consisting of a mix of angular fragments of quartz, feldspar, and mafic minerals set in a muddy base (commonly called a "dirty sandstone or mudstone" because of its mixed size fractions). Graywacke is the general term applied to sediments deposited by turbidity flows, and they commonly show graded bedding. Graywacke is a common rock-type in the Coast Ranges of California and other active continental margin regions around the world. It is exposed on land where tectonic forces push up rocks that originally formed in the deep ocean (examples in Figures 6-65 and to 6-66).
Turbidites are sedimentary deposits associated with turbidity flows—they commonly appear as interbedded layers of sandstone and shale. Conglomerate typically occurs in thicker beds and were originally deposited as gravel and mud on ancient submarine fans closer to the mouths of submarine canyons or in channels carved into the seabed. |
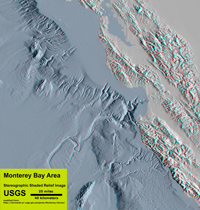 |
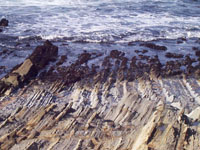 |
Fig. 6-65. Turbidity currents scour submarine canyons in the deep offshore environment and deposit sediments in the deep ocean. Deep-sea fans build up the continental rise region at the base of the continental slope and spread for hundreds of miles seaward, sometimes extending onto abyssal plains. |
Fig. 6-66. Cretaceous-age turbidites (turbidity current deposits) at Bean Hollow State Beach, California. Each layer represents an undersea "storm" (turbidity flow) that spread across a deep sea fan on a continental rise. They were pushed up by tectonic uplift along the coast. |
6.22
Abyssal Clays
Abyssal clays are
very fine-grained sediments, mostly clay minerals and iron-rich mineral dust that are mostly blow in by the wind from distant terrestrial sources. Much of the abyssal clay components are derived from dust storms in the world's desert regions and from explosive volcanic eruptions that can blow fine particles high into the atmosphere. Abyssal clays are also fine-grained material carried and redistributed by ocean currents such as tail end of far-turbidity currents that can travel hundreds to even thousands of miles away from continental margins. Abyssal clays in the deep ocean basins accumulate very slowly relative to other ocean sediments. Some of the fine-grained material can possibly be from cosmogenous sources in some locations. Abyssal clays dominate sediments on the seafloor in the northern Pacific Ocean basin (see discussion on volume and distribution of marine sediments (in Figure 6-18 above). |
6.23
Biogenous Sediments in the Marine Environment
Biogenous sediments include sediments formed by accumulation of organic materials. Biogenous sediments are mostly composed of the remains of organisms (including skeletal remains of microplankton (both plants and animals), plant remains (wood, roots, and leaves) and remains of larger animals including shells of invertebrates, such as shells, coral fragments, and fish and other vertebrate teeth, bone, and scales, and fecal material left behind by any type of organism. Biogenous sediments may be partly mixed with lithogenous sediments (continental-derived sediments) in coastal regions, particularly where streams and rivers contribute sediments.
Bioaccumulation is the buildup of organic remains, such as deposits associated with coral reefs, shell or bone beds, and algae and ooze (calcareous and siliceous). On land bioaccumulation in swampy environments produces peat beds (with burial and time, peat eventually can be converted to coal). In many passive margin regions in tropical regions, carbonate sediments form and accumulate forming massive deposits along continental margins.
Carbonate Reefs
A reef is a general name for a ridge of jagged rock, coral, or sand just above or below the surface of the sea. A carbonate reef is one that is made of skeletal material composed of coral, coralline algae, and other carbonate skeletal material. Carbonate reefs are commonly called coral reefs but not all organisms that look like corals are actually corals—other organisms that create solid structure (branching or not) include coralline algae, bryozoans, sponges, stromatoporoids, and many other types of invertebrates). Figure 6-67 illustrates the variety of settings and features associated with carbonate depositional environments.
Carbonate (coral) reefs form in clear shallow, warm, tropical marine waters.
Over time, lime sediments are produced by biological activity in and around carbonate reefs. Carbonate reefs grow at rates of 10-30 feet per thousand years. Wave action and currents will erode and redistribute lime sediments offshore where it may accumulate, slowly building up massive carbonate platforms (becoming regions underlain by limestone). Examples of carbonate platform regions include the Bahamas, South Florida, and the Yucatan Peninsula (Figure 6-68 and 6-69).
The world's largest reef system is the reef tracts, islands, and tidal shoals associated with the Great Barrier Reef located along the east coast of Australia (Figure 6-70). The Great Barrier Reef is composed of over 2,900 individual carbonate reefs and about 900 islands stretching for over 1400 miles (2,300 km) along the northeast coast of Australia and encompassing about 133,000 square miles (344,400 km2). It is the largest feature of biological origin on Earth. Similar reef tracts have formed throughout geologic history in other locations around the world.
|
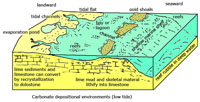 |
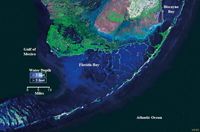 |
Fig. 6-67. Carbonate depositional environments include coral reefs, keys, shoals, tidal flats, bays, and other coastal and offshore features. |
Fig. 6-68. South Florida is part of a growing carbonate platform with the Keys consisting of an ancient and modern forming a barrier reef complex. |
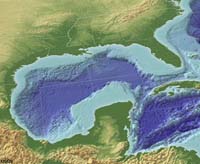
Fig. 6-69. Carbonate platforms surround much of the Gulf of Mexico. They include continental shelf regions around the Yucatan Peninsula, South Florida, and islands of the Caribbean where Biogenous sediments form and accumulate. |
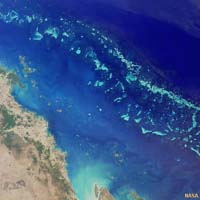
Fig. 6-70. Great Barrier Reef - The world's largest organic deposit. The growth of the great reef tract has kept pace with the global rise in sea level since the end of the Wisconsinian ice age. |
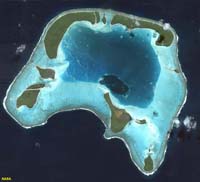 |
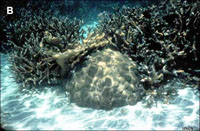 |
Fig. 6-71. Atolls are volcanic islands or seamounts covered or surrounded by fringing carbonate reefs that build up even long after the volcano stopped erupting. |
Fig. 6-72. Lime mud and sand accumulating around a living coral reef. Fine limey sediments are created mostly by organisms feeding on other reef organisms. |
6.24
Limey Sediments and Limestone
Lime mud is sediment composed of calcium carbonate (CaCO3) derived from the skeletal remains of shelled organisms, coral, and calcareous algae and plankton. Large amounts of lime mud is created by waves battering reefs and reef organisms (including dead corals and other calcareous skeletal material) being chewed up and excreted by reef-living organisms (Figures 6-72). With compaction and cementation (lithification) limey sediments become limestone (Figure 6-73).
Limestone is a sedimentary rock consisting predominantly of calcium carbonate (CaCO3); the rock must have >50% calcium carbonate to be considered a limestone. Some limestones preserve large quantities of fossil material as crushed up shells or even old reef communities are sometimes preserved in nearly intact orientation of the corals and other calcareous organisms. These organic remains are made up of tiny crystals of two mineral forms of CaCO3—calcite and aragonite. Aragonite is more soluble and is chemically less stable, and will usually convert to calcite with time.
Most limestone exposed throughout the United States formed in ancient shallow marine seaways that flooded portions of the continent in the geologic past. Large regions within the United States are underlain by thick sequences of limestone rock formations representing all geologic time periods from Precambrian age to the present (Figure 6-74). In many locations the limestone beds are many thousands of feet thick. Most caverns form in limestone. Sinkholes form in limestone regions (See Sinkholes [USGS])
Limestone is commonly used in the manufacture of lime for cement, used as building stone, and used to manufacture steel and many other products. Ancient carbonate deposits contain some of the world's largest petroleum reserves. |
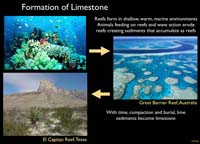
Fig. 6-73. Skeletal remains of calcareous reef organisms erode and accumulate over time. "Limey" (shallow and warm) depositional environments are where lime sediments accumulate. Lime sediments turn to limestone.
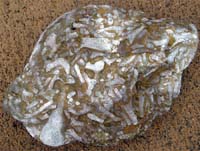
Fig. 6-75. Fossiliferous limestone. This sample from Ohio is loaded with ancient coral-like fossils called bryozoans (not corals). |
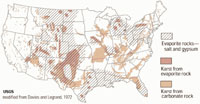
Fig. 6-74. Map of the United States showing the location of carbonate rocks in the subsurface. Limestone rock formations occur under about 40% of the continental United States!
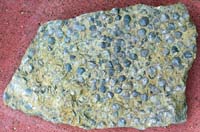
Fig. 6-76. Fossiliferous limestone. This layer from Cincinnati, Ohio is loaded with ancient brachiopod shells that accumulated in an ancient inland seaway. |
6-25
The oceans are full of many varieties of microscopic organisms, but only several varieties are responsible for generating vast quantities of biogenous sediments.
Ooze is slimy mud sediment (soft and mushy) on the bottom of an ocean or lakebed formed from the accumulation of skeletal and organic remains of microscopic organisms (phytoplankton and zooplankton).
• Oozes can be dominantly calcareous or siliceous in composition.
• To be considered an "ooze" sediment must consist of >30% biogenous material (Figure 6-77).
• Oozes form slowly - accumulating at a rate of 1/2 to 2 1/2 inch per 1000 yrs.
• Oozes form in low energy environments and are very fine grained (clay sized particles).
|
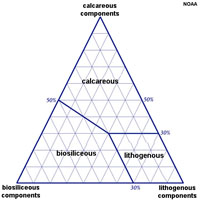 |
Fig. 6-77. Components of oozes: calcareous, biosiliceous, and lithogenous materials |
6.26
Calcareous oozes
Calcareous oozes are sediments dominantly composed dominantly of calcium carbonate (CaCO3). Two dominant groups of microorganisms that contribute carbonate remains: Coccolithopores (phytoplankton) and Foraminifera (zooplankton)
|
Coccolithopores
Coccolithopores are single-celled marine phytoplankton (microscopic plants) that live in large numbers throughout the upper layers of the ocean. Unlike any other plant in the ocean, coccolithopores secrete shells of microscopic plates made of calcite (CaCO3). These scales, known as coccoliths, are shaped like hubcaps and are only three one-thousandths of a millimeter in diameter (Figure 6-78). Coccolithopores are part of base of the food chain and contribute vast quantities of coccoliths as sediment to large regions of the ocean basins. Coccoliths are concentrated in calcareous ooze.
Coccoliths first appear in the fossil record in Triassic time. Because they are composed of low-magnesium calcite (the most stable form) they are easily fossilized and preserved in sedimentary rocks.
What is a Coccolithopores? (NASA)
|
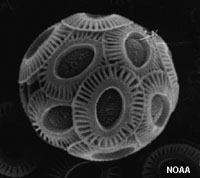 |
Fig. 6-78. A Coccolithopores is covered with calcareous plates called coccoliths. |
6.26
Foraminifera (Forams)
Foraminifera (or forams) are a large group of single-celled zooplankton, most species have calcareous shells (or tests). Their shells are commonly divided into chambers which are added during growth and form patterns including spirals, open tubes, or hollow spheres (Figure 6-79). Depending on the species, the shell may be made of crystalline calcite, organic compounds, or sand grains and other particles cemented together. They are usually less than 1 mm in size, but some species grow much larger, reaching up to 20 cm. The majority of foraminifera species are benthic (meaning they live on or within the seafloor sediment) while typically smaller varieties are floaters (planktonic) in the water column at various depths. Foraminifera are found in all depths of the ocean, although deep ocean varieties do not have calcareous tests. They contribute a significant volume of sediments to carbonate reefs and a major component of carbonate oozes throughout ocean basins.
Over 10,000 species are recognized, both living and fossil. They first appeared in the fossil record in Cambrian time. |
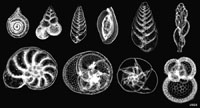 |
Fig. 6-79. Examples of foraminifera tests. |
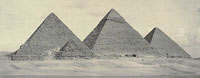 |
Fig. 6-80. Pyramids of Giza, Egypt are constructed with foraminiferal limestone. |
6.27
Calcium carbonate compensation depth (CCD)
Calcareous sediments are fairly evenly distributed in oceans, but their occurrence is influenced by the solubility of calcium carbonate. Calcium carbonate forms and is stable in shallow, warm seawater, but it will dissolve in cold seawater. Carbon dioxide dissolves easily in cold water, so CaCO3 will dissolve in cold water. The calcite compensation depth (CCD) is the depth in the oceans where the rate of calcium carbonate material forming and sinking is equal with the rate the material is dissolving. Below the CCD no calcium carbonate is preserved—generally there is no CaCO3 beneath about 15,000 feet (4500 meters) (Figure 6-81).
Skeletal remains composed of calcium carbonate (CaCO3) sinking into the deep ocean are mostly microscopic plankton. As carbonate materials settle or are moved by currents in to deep water, the smallest fragments dissolve before larger, denser fragments. The lysocline is the depth at which CaCO3 begins to dissolve rapidly.
|
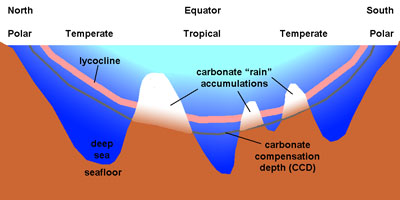 |
Fig. 6-81. Relationship of the lysocline and the carbonate compensation depth (CCD) relative to depth of the ocean and latitude. The lysocline and CCD are at the surface near the poles where the water is cold. Calcareous oozes accumulate only above the CCD. |
6.28
Chalk
Chalk is a soft, fine-grained, white to grayish variety of limestone that is composed of the calcareous skeletal remains of microscopic marine organisms including coccoliths and foraminifera. Some of the purest varieties can have up to 99 percent calcium carbonate (see Figure 6-82). The White Cliffs of Dover, England are one of the most iconic landscape features in the United Kingdom. The White Cliffs consist of Cretaceous-age chalk deposited about 89 to 85 million years ago in more tropical conditions than exist in the region today. The layers of chalk reach nearly 500 meter thick. The sediment the chalk formed from was coccolithopore ooze. |
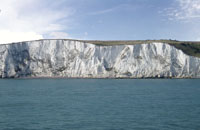 |
Fig. 6-82. The White Cliffs of Dover, England are chalk. |
6.29
Siliceous oozes
Siliceous oozes are sediments dominantly composed dominantly of SiO2 (silica).
Two dominant groups of organisms that contribute siliceous remains: diatoms and radiolarians.
Diatoms
Diatoms are the most common plankton. Diatoms are phytoplankton (single-celled microscopic marine plants).
• Diatoms are most common in polar regions, but are also know from tropical and subtropical regions as well.
• Very important for upwelling nutrients (where deep water rich in .
• Diatoms have many economic uses including in beer filters, pool filters, and optical glass. |
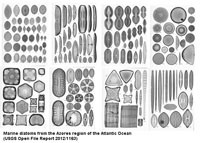 |
Fig. 6-83. Example of diatoms. These are images taken with a microscope. |
6.30
Radiolarians
A radiolarian is a single-celled aquatic animal (zooplankton) that has a spherical, amoeba-like body with a rigid spiny skeleton of silica. There are hundreds of known species of radiolarians (See a list on radiolaria.org website).
Figure 6-85 is a photomicrograph depicting the siliceous tests of ten species of marine radiolarians.
Upon death, their tests can accumulate on the seafloor and form siliceous marine sediments known as radiolarian ooze (a form of siliceous ooze). Radiolarians first appear in the geologic record in early Cambrian time and have experienced several periods of proliferation and extinctions as recorded in the geologic record.
Today,
radiolarians are more common in equatorial regions.
|
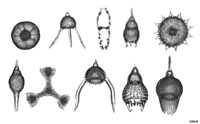 |
Fig. 6-84. Example of Radiolarian skeletons (tests). These are images taken with a microscope. |
6.31
Chert
Chert is a fine-grained siliceous sedimentary rock. It is a hard, dense, and consist chiefly of interlocking microscopic crystals of quartz and may contain opal. It has a conchoidal fracture and may occur in a variety of colors. Most chert forms from recrystallization of siliceous microplankton remains (siliceous ooze eventually looses its water content, recrystallizes and turns into chert.
Organic residues preserved as chert beds are known from rocks dating back to early Precambrian time. Banded-iron formations (BIFs) are composed of interbedded layers of iron-oxide minerals and chert, and are thought to be biogenous in origin. Younger marine cherts are mostly formed from diatoms and radiolarian oozes. |
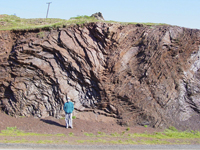 |
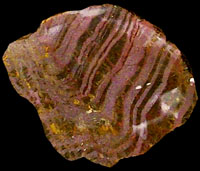 |
Fig. 6-85. Layers of marine chert exposed in the Marin Headlands, California |
Fig. 6-86. Banded-iron formation (with chert) from the Precambrian era. |
6.32
Sedimentary Rock Formations
A rock formation is the primary unit of stratigraphy, consisting of a succession of strata useful for mapping or description. A rock formation typical consists of a unique lithology (rock type) that has a relatively defined geologic age and is considered mappable (occurs throughout area or region, both on the surface and in the subsurface. Rock formations can be of igneous, sedimentary, or metamorphic origin. Sedimentary rock formations preserve information (including fossils and sedimentary structures) about the sedimentary environments they formed in. Figures 6-87 to 6-89 are examples of marine sedimentary rock formations.
|
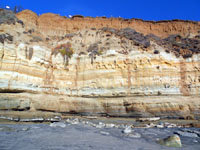 |
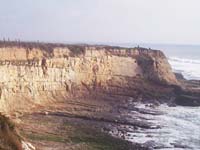 |
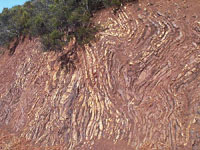 |
Fig. 6-87. Ancient beach, bay, and coastal dune deposits exposed in rock formations at the Del Mar Dog Beach, San Diego County, California |
Fig. 6-88. Ancient continental shelf deposits preserved in the Santa Cruz Mudstone Formation (Miocene-Pliocene age) in Santa Cruz, CA |
Fig. 6-89. Ancient deep ocean siliceous ooze deposits preserved as ribbon chert in the Franciscan Formation (Jurassic age), Santa Cruz Mountains, CA |
6.33
Finally, food for thought...
Nuclear bomb testing and nuclear power-plant disasters has created a new identifiable sediment boundary preserved in Holocene sediments worldwide. This boundary is now associated with a proposed new epoch of the Quaternary Period called the Anthropocene — when human activities became plausibly the dominant force causing changes to Earth's global physical environment.
The United States conducted surface nuclear testing at Bikini Atoll in the South Pacific. Testing took place between 1946 and 1958. During that time, 23 nuclear devices were detonated at seven test sites on the reef itself, on the sea, in the air and underwater. So far, 8 nations in 2016 have successfully tested nuclear weapons. Whereas the last atmospheric nuclear test was in 1980, underground testing has continued.
Another proposed epoch name, not yet adopted, is perhaps.... Weshouldhavecene. |
Fig. 6-90. H-bomb test destroys Bikini Atoll |
|