Introduction to Physical Geology |
|
Chapter 14 - Ocean Basins |
14.1
Despite being the 21st century, Earth's ocean basins remain mostly unexplored in any detail. Oceans cover more the 70% of the Earth's surface. Despite the difficulty of exploring of exploring the deep, dark expanse of the world's oceans, the world's scientific community has collaborated to collect and release information about the nature and origin of ocean basin features.
This chapter describes the major physiographic features that occur beneath the oceans from the world's coastlines to the deep expanse of the ocean basins. Much of what is presented in this chapter expands on concepts presented in earlier chapters.
This chapter also reviews and expands on the origin of the world's ocean basin through Plate Tectonics Theory (introduced in Chapter 6). Discussions focuses on characteristics of ocean crustal rocks formed along mid-ocean ridge spreading centers and the unique types of marine sediments that are found forming around and within ocean basins.
|
Click on thumbnail images for a larger view. |
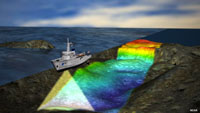
Fig. 15-1. Ship equipped with sonar equipment scanning the seamounts. |
14.2
Ocean Basin Mapping
Bathymetry is the measure of depth of water in oceans, seas, lakes, and rivers. Bathymetry data is used to create maps (called charts) of the seamounts. Bathymetric charts are the equivalent of topographic maps on land.
In the past, the depth of water was
measured by lowering weighted lines overboard. Mapping in this crude manner was mostly limited to shallow waters along coastlines and port harbors. The development of echolocation began during World War 1and was advanced to sonar in the 1920s. SONAR (short for SOund NAvigation & Ranging) is a system for detecting objects under water and for measuring the water's depth by emitting sound pulses and detecting or measuring their return after being reflected off an object or the seamounts (Figure 15-1).
Advances in submarine and marine warfare during World War 2, and especially the development of advanced nuclear submarines in the 1950s during the Cold War, created an intense demand for more accurate maps of the world's ocean basins. Information from US Navy investigations combined with information from commercial transatlantic communications cable-laying operations were used to create the first details maps of the North Atlantic ocean basin was released in 1957 (Figure 15-2). The first map of the world’s ocean basins released to the public was in 1977—these maps ultimately served to initiate research and interpretations leading to Plate Tectonics Theory.
Deep-Sea Drilling and Geologic Mapping of the Seafloor
Starting in the late 1960s, the US National Science Foundation and several foreign countries collaborated with funding the Deep Sea Drilling Project (DSDP) operated Scripps Institution of Oceanography. The project used the deep sea drilling ship, the D/V Glomar Challenger. Between 1968 to 1983, this exploration ship drilled 1,124 holes at 624 locations worldwide (Figure 15-3). In addition to drilling and collecting core samples of sediments and rocks, the ship collected geophysical data from the wells and the surrounding seafloor. The data from the ship's activities, and from many other oceanographic investigations since then, have been fundamental to resolving questions about the geology and origins of the world's ocean basins.
More modern methods used by the US Navy (and other organizations) use side-scan sonar imagery and sub-bottom profiler data to map and interpret the geography and geology of the seafloor. The side-scan sonar instrument is towed below the surface behind a ship and emit high-frequency sound pulses which reflect off the sea bed. This data is used to compile black-and-white map-like images of the seafloor. Sub-bottom profilers emit low-frequency sound pulses which can penetrate into the seafloor. Sound pulses of returning signals from the seabed are captured as the ship moves along, gradually creating a profile of the geology directly below the ship. In addition, sediment samples and photographs taken with cameras on submersible vehicles are used to produce geologic maps of the seafloor. Satellite orbital motions are impacted by subtle differences in the Earth's gravity around such features as mountains or volcanoes on the bottom of the ocean. The Navy used satellite orbit data to compile the first gravity map of the seafloor (Figure 15-4). |
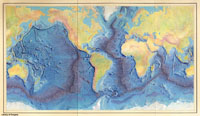
Fig. 15-2. First published map of the worlds ocean basins and land surface published by Marie Tharp, Bruce Heezen, and Heinrich Berann in 1977.
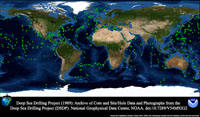
Fig. 15-3. Map showing locations where the Glomar Challenger drilled deep sea wells to sample sediments and rocks from the seafloor.
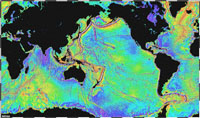
Fig. 15-4. Map of the seafloor produced by gravity measurements from satellite data. |
14.3
Geologic Map of the Ocean Floor
Collaborative efforts between numerous science organization and governments have led to the first complete geologic map of the world's ocean basins (Figure 15-4). The figure shows the age of rocks on the seafloor in a color spectrum with red being the youngest rocks (recently formed to several million years) and blue being the oldest (about 200 million years).
Ocean crustal rocks older that 200 million years have either already sunk into the mantle or have been "modified" by metamorphic and igneous processes, and pushed up and accreted onto continental margins bordering the ocean basins. Rocks grow progressively older in age as they slowly move away from spreading centers along mid-ocean ridges. Ancient ocean crust is sinking back into the mantle along subduction zones associated with deep sea trenches—such as along the margins of the Pacific Ocean (discussed below). In contrast, the relatively smaller Atlantic Ocean basin is expanding (slowly growing wider a few inches each year). This motion is pushing continents on either side of the Atlantic farther apart, and subduction zones have not yet formed along the margins of the Atlantic Basin. It could be argued that the Pacific Ocean basin is shrinking at the expense of the expanding Atlantic and Indian Ocean basins. |
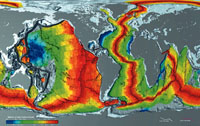
Fig. 15-4. Map showing the geologic age of the seafloor of the world's oceans. Red are youngest rocks and blue are the oldest. |
14.4
Major Provinces of the Ocean Floor
Ocean basins are subdivided into 3 general provinces, each having unique physical and geologic characteristics:
• Continental margins - these are regions that extend from the coastlines across shallow submarine shelf regions to just beyond the edge of continents where the seafloor descends into deep water (Figure 15-5).
• Deep-ocean basins - This includes parts of the oceans where deep water prevails. Deep ocean basins cover the greatest portion of the Earth's surface.
• Oceanic (mid-ocean) ridges - nearly 12,000 miles of mountain belts run through ocean basins and are associated with divergent plate boundaries (spreading centers).
Each of the major provinces are discussed in detail below. |
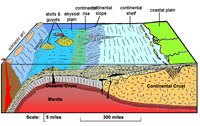
Fig. 15-5. Submarine landscape features associated with a continental margin to deep-ocean basin. |
14.5
Continental Margins
Continental margins border continental landmasses are submarine geographic regions located between the shoreline and deep ocean. They are the submerged edge of continents. Continental margins include subregions and submarine geographic features:
• continental shelf
• continental slope
• continental rise
• submarine canyons
Figure 15-6 illustrates a submarine map of a portion of a continental margin near Monterey Bay, California that illustrates subregions of the continental margin. Brown area is land (between the Big Sur to the south and Santa Cruz to the north), orange represents the submerged shallow continental shelf, and increasingly darker shades of blue show increasing water depths into the deep ocean along the continental margin. Monterey Canyon and other submarine canyons cut into the continental slope. A seamount is in the lower left.
Continental margins are influenced by continental processes including regional tectonic uplift and subsidence, and erosion and deposition.
|
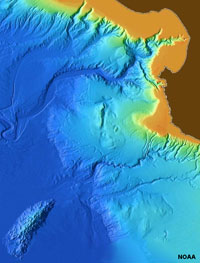
Fig. 15-6. Bathymetric view of Monterey Canyon and other seafloor features along the central coast of California. |
14.6
Active vs. Passive Continental Margins
Continental margins typically fall into two classes: active and passive. North America is partly surrounded by both active and passive continental margins (Figure 15-7).
An active continental margin is a coastal region that is characterized by mountain-building activity including earthquakes, volcanic activity, and tectonic motion resulting from movement of tectonic plates. Characteristics of active continental margins include:
• They are located mostly along convergent plate boundaries.
• In most places along active margins, the continental slopes descend abruptly into a deep-ocean trench (there may be no continental rise), or they may be rugged regions associated with large fault systems.
• Most are located primarily around the margins of the Pacific Ocean (the Ring of Fire).
Active continental margins are characterized by rugged coastlines with narrow beaches and steep sea cliffs. The West Coast of the United States is an active continental margin (Figure 15-8).
Passive continental margins occur where the transition between oceanic and continental crust which is not an active plate boundary. Examples of passive margins are the Atlantic and Gulf coastal regions which represent setting where thick accumulations of sedimentary materials have buried ancient rifted continental boundaries formed by the opening of the Atlantic Ocean basin.
Passive continental margins are characterized by wide beaches, barrier islands, broad coastal plains. The Atlantic Coast of the United States is a passive continental margin (Figure 15-9).
Figure 15-10 is a comparison of general geographic and geologic features on active and passive continental margins. shoreline features associated with continental margins are discussed in Chapter 16). |
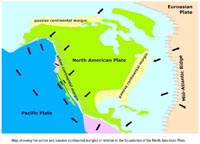
Fig. 15-7. Active and passive margins of North America. The East Coast and North Slope are now passive margin regions located within the greater North American Plate. |
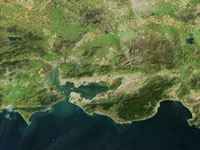
Fig. 15-8. Active margin: San Francisco Bay and Monterey Bay region has actively rising coastal range mountains and sinking coastal basins. |
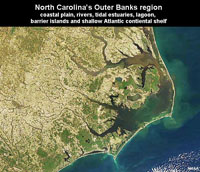
Fig. 15-9. Passive margin: North Carolina's Outer Banks region showing coastal plain, rivers, tidal estuaries, lagoon, barrier islands, and shallow Atlantic continental shelf. |
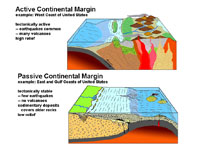
Fig. 15-10. Comparison of active and passive continental margins. Passive margins are on the trailing edge of a moving continental landmass. |
14.7
Continental Shelves
A continental shelf is a submerged nearshore border of a continent that slopes gradually and extends to a point of steeper descent to the ocean bottom. Continental shelves are submerged extension of the continent.
Continental shelves typically have
low relief: they usually have less than 1 degree of slope. Average is about one tenth of one degree.
Continental shelves are influenced by a variety of geologic processes, particularly associated with the erosion and deposition of sediments on beaches, deltas, and carbonates (coral reefs). Shallow water coastal and shelf environments are particularly influenced by the impact of large storms.
Continental shelves are commonly cut by submarine canyons.
Continental resources are areas with important natural resources, particularly fisheries, but also oil and gas, and sand and gravel.
During the peak of the last ice age, the world's continental shelves were mostly exposed coastal plain environments.
A shelf break is a general linear trend that marks the boundary between the relatively flat continental shelf and the drop off into deeper water on the continental slope. The shelf break generally follows the ancient shorelines that existed at the peak of the continental glaciation periods of the ice age when sea level was as much at 400 feet (120 meters) lower that present sea level.
|
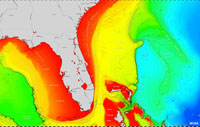
Fig. 15-11. The continental shelf around Florida (shown in red) gradually transitions to the continental slope (yellow and green). Florida displays features of a typical "passive continental margin" having wide coastal plains, wide continental shelves, and gentle slopes extending into deep water. |
14.8
Continental Slopes
A continental slope is the slope between the outer edge of the continental shelf and the deep ocean floor. The continental slope is cut by submarine canyons in many locations. The continental slope marks the seaward edge of the continental shelf.
Continental slopes typically follow the
boundary between continental crust and oceanic crust (Figure 15-12).
Continental slope range in steepness from 1 to 25 degrees, average is 4 degrees.
• Pacific (active margin) average >5 degrees.
• Atlantic (passive margin) average about 3 degrees
Continental slopes are cut by submarine canyons. The dominant process influencing slopes are sediment deposition and erosion by turbidity currents (discussed below).
|
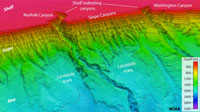
Fig. 15-12. The continental slope off the coast of Virginia is cut by numerous submarine canyons that drain sediments to the continental rise at the base of the slope. |
14.9
Continental Rises
A continental rise is a wide, gentle incline from a deep ocean plain (abyssal plain) to a continental slope (Figure 15-13).
A continental rise consists mainly of silts, mud, and sand, deposited by turbidity flows, and can extend for several hundreds of miles away from continental margins. Although it usually has a smooth surface, it is sometimes crosscut by submarine canyons extending seaward of continental slope regions.
The continental rise is generally absent in regions where deep-sea trenches exist where subduction zones are active.
Continental rises feature deep-sea fans. In appearance they are much like alluvial fans on land found along the fronts of mountain ranges. Deep-sea fans are accumulations of sediment deposited by turbidity currents (called turbidites) at the foot of the continental slope (discussed more in section 14-25 below). Turbidites are underwater landslide deposits. Over time they build up the large deep-sea fans that coalesce to form a continental rise along some continental margins. |
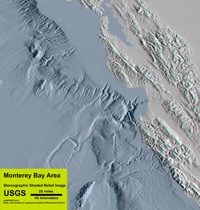
Fig. 15-13. Bathymetry of the Monterey Bay offshore region highlights the character of the continental slope and rise. |
14.10
Submarine Canyons
Submarine canyons are similar to river gorges carved in mountainous regions on land, however they tend to be both much larger and deeper (Figure 15-14). Characteristics of submarine canyons include:
• They generally form perpendicular to coastline.
• They are commonly associated with zones of weakness such as a fault or a drowned river valley (flooded by sea-level rise).
• They start on continental shelf and cut into (erode) shelf and upper slope, commonly near the mouth of a bay or river.
• They are carved by undersea erosion processes associated with turbidity currents. Turbidity currents transport sediment into deep ocean basins via submarine canyons.
• Turbidity currents moving down submarine canyons eventually slow down and deposit sediments on the continental rise as deep-sea fans on continental rise regions. Submarine fans accumulate below the mouths of submarine canyons.
|
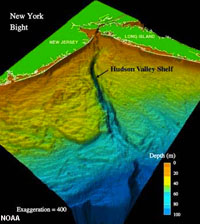
Fig. 15-14. Hudson Canyon offshore of New York City |
14.11
Deep-Ocean Basins
Deep-ocean basins cover the greatest portion of the Earth's surface. Geographic features associated with deep-ocean basins include trenches, abyssal plains, ocean ridges and rises, and submarine mountainous regions.
Abyssal Plains
An abyssal plain is an underwater plain on the deep ocean floor, usually found at depths between 4500 and 6000 meters that extends from the continental rise (continental lithogenous sediments accumulate along continental margins) to the distant deep ocean basin where continental-derived sediment deposition is not significant.
Abyssal plains are large horizontal seafloor regions - typically some of the flattest places on the Earth's surface (on the seafloor, Figure 15-15). Abyssal plains are the largest physiographic regions on the planet, covering millions of square miles in ocean basins.
Abyssal plains are underlain by oceanic crust that formed and moved away from spreading centers associated with mid-ocean ridges and rises. Because they are so far from land they have
very slow sedimentation rates. Some places receive less than 1 centimeter per 1000 years. The dominant geologic process is planktonic rain which blankets seafloor with organic sediments called oozes (discussed below).
|
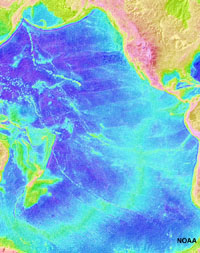
Fig. 15-15. Abyssal plains dominate the Pacific Ocean basin. |
14.12
Trenches
Trenches are
long, relatively narrow canyon-like features that run parallel to continental margins.
They are the deepest parts of ocean basins. Most trenches are located in the Pacific Ocean - the deepest is the Marianas Trench—10,994 meters (36,070 feet) (Figure 15-16).
Trenches occur where
mobile lithospheric plates plunge into the mantle (subduction zones). Ocean crust is in contact with cold ocean water. Over time ocean crust cool and shrinks, increasing in density. Along zones where crustal plates are colliding, the old, cold oceanic crust sinks back into the mantle. Along the way, it heats up, and water and gases trapped in the rock escapes and works its way back to the surface, helping to melt rocks along the way. As a result, volcanic island arcs associated with trenches are are locations of intense volcanic activity above the descending side of the subducting plate sinking back into the mantle. Subduction zone faults are associated with trenches and produce most of the most powerful earthquakes on the planet. |
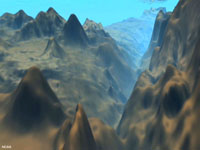
Fig. 15-16. An animated view of part of the Marianas Trench. See a NOAA animation. |
14.13
Seamounts, Islands, Atolls, and Guyots
A seamount is any isolated mountain-sized feature that rises above the seafloor. A seamount may be a large tectonic block that separated from a large continental landmass or may be an ancient or even active submarine volcano. A submarine mountain that is partly exposed above the ocean surface is called an island. Many seamounts (and islands) are isolated volcanic peaks rising off seafloor. Many are part of mid-oceanic ridges or associated with oceanic hotspots (discussed below). For instance, the Hawaiian Islands are part of the Emperor Seamount Chain (see Figure 15-18). The South Pacific region is a region with numerous seamounts, of which many are islands, atolls, or guyots (Figure 15-19 and 15-20).
An atoll is a ring-shaped reef, island, or chain of islands formed of coral, typical on a foundation of an extinct volcano in the ocean. The limestone ring forms along the margins of the volcano. Over time, the volcano either erodes away or sinks below the surface, but the limestone rim continues to grow and expand over time. A guyot is a submarine mountain (seamount) with a flat top. Most guyots are ancient submarine volcanoes that have been beveled by wave action before sinking into ocean depth and may lack the fringing limestone reefs associated with atolls.
|
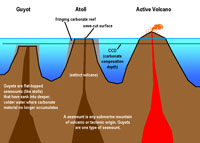
Fig. 15-17. Formation of ocean-basin volcanoes, atolls, and guyots. Guyots are flat-topped seamounts that have sunk deep enough into cold water so that reefs cannot form. |
14.14
Fig. 15-18. Hawaii is the youngest volcanic island of the Emperor Seamount Chain. Many of the older seamounts were once volcanic islands but are now atolls or guyots.
|
Fig. 15-19. The South Pacific region has numerous islands, seamounts, atolls, and guyots. Chains of undersea volcanoes formed above plumes of mantle material rising beneath the northwest moving Pacific Plate. |
Fig. 15-20. A satellite view of an atoll displaying a fringing carbonate reef platform (with islands) surrounding an eroding central volcanic peak. Most atolls of the world are located in tropical regions of the South Pacific and Indian Oceans. |
Fig. 15-21. Seamounts and islands offshore of southern California. Many of these features are associated with active faults that are moving great blocks of crust across the seafloor. |
14.15
Oceanic (Mid-Ocean) Ridges and Rises
Mid-ocean ridges (MORs) are broad, linear swells along divergent plate boundaries in ocean basins (Figure 15-22). They are associated with extensive faulting and small earthquakes, and along their crest there is high heat flow form new crust forming from the cooling of molten material derived from the mantle and remelting of the crust.
Mid-ocean ridges are present in all ocean basins. They are the shallowest and youngest parts of deep ocean basins.
Features associated with ocean ridges include volcanism (undersea volcanic features), undersea rift valleys, and hydrothermal vents.
As newly formed ocean crust moves away from spreading centers, the crust gets older (and colder and denser) and sinks deeper into the ocean basin (isostatically adjusting as the lithosphere settles deeper into the asthenosphere). In addition, layers of sediments thicken away from the mid-ocean ridges.
|
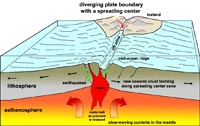
Fig. 15-22. Formation of new oceanic crust along a spreading center associated with a mid-ocean ridge. Some spreading centers appear on land. For example, a portion of the Mid-Atlantic Ridge is exposed on Iceland. |
14.16
What is the difference between a mid-ocean ridge and a mid-ocean rise?
The two features are basically the same except for their shape (topography) and how fast they form.
Topographic differences between ridges and rises are controlled by spreading rates.
• Ridges are steeper with slow spreading rates (1-5 centimeters per year) - Example: Mid-Atlantic Ridge.
• Rises are flatter with fast spreading rates (greater than 9 centimeters per year) - Example: East Pacific Rise (Figure 15-23).
Why are ridges and rises so high?
• Newly created oceanic lithosphere is hot and occupies more volume (less dense) than cooler older rocks.
• As the oceanic crust travels away from the ridge crest it cools and becomes more dense and sinks. |
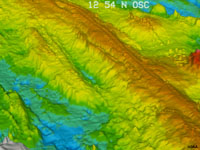
Fig. 15-23. Bathymetric image of the spreading center rifts on the East Pacific Rise. |
14.17
Vents on the Seafloor: Black Smokers, White Smokers, and Deep-Sea Vent Communities |
Hydrothermal vents form where there is volcanic activity on or below the ocean floor, such as along the mid-ocean ridges (Figure 15-24). Water seeps through cracks in the seafloor and is heated by hot rock deep below the ocean crust to as high as 400°C. This hot water is under too much pressure to boil, but it erupts as smoky fountains at vents on the seafloor. The hottest vent produce unusual chimney-like towers called black smokers (Figures 15-25 to 15-27). The hot water contains dissolved metals (including iron, manganese, zinc, copper, sulfur, and others). When they encounter the cold ocean water, the minerals precipitate, making dark plumes of water and irregular deposits (including “chimneys”) on the seabed.
Cooler vents produce white smokers that are dominated by deposits of calcium-rich minerals, including anhydrite gypsum (CaSO4) and calcite (CaCO3). Minerals in ocean crustal rocks are rich in calcium, which dissolves easily on cold seawater. Where the warmed seawater rises back to the surface, the calcium-enriched water produces white smokers along with deposits of minerals that also host deep-sea bed communities (Figures 15-28 and 15-29).
Deep-Sea Vent Communities
Deep-sea vents have been identified in many locations along mid-ocean ridges and along the flanks of undersea volcanoes. The rich supply of nutrients support chemotrophic bacteria (feeding on sulfur compounds) that support a complete food web of seafloor creatures, including tube worms, arthropods, fish, and other benthic life forms adapted to these harsh and temporary environments (Figures 15-30 and 15-31). Current thought is that many of the major economic mineral deposits of the world may be associated with ancient deep-sea vent deposits. |
|
|
|
|
Fig. 15-24. Deep-sea vents form from seawater convection in ocean crust. |
Fig. 15-25. Mineral-rich black smoker deposits are rich in copper, zinc, iron, and others |
Fig. 15-26. Black smokers venting very hot mineral-rich water on the seabed. |
Fig. 15-27. Black smokers with a deep-sea community around them. |
|
|
|
|
Fig. 15-28. White smokers with chimneys of the seabed. |
Fig. 15-29. White smokers with chimneys of the seabed. |
Figure 15-30. Deep-sea vents host rich benthic communities |
Fig. 15-31. Deep-sea vent community. |
|
14.18
Origin of Oceanic Lithosphere and Basins
At mid-ocean ridges (spreading centers), newly forming lithospheric plates move apart. This creates space for magma to flow upward into the fractures forming along rift zones. Over time, more and more fractures form, they fill with magma, and then cool and fracture making pathways for more magma to flow upward and the process is repeated. This process generates new oceanic lithosphere (ocean crust). In some locations around the world, large mountain-sized blocks of old ocean crust has been pushed up along faults and exposed on land—where they are exposed, they are called an ophiolite sequence because they consist of unique varieties of rocks that formed in a sequential order (Figure 15-32).
Formation of Oceanic Lithosphere
Zones of active rifting along mid-ocean ridges (spreading centers) are typically 20 to 30 km wide. In some locations, the very hot, fluid lava migrating upward from the asthenosphere (upper mantle) reaches the surface of the seafloor resulting in formation of undersea volcanoes. These undersea eruptions produce pillow basalts - pillow-shaped pods of basalt rock formed where the hot lava cools rapidly when exposed to seawater.
As new lithosphere forms, older ocean crust gradually moves away from the mid-ocean ridge crest beyond the zone of active rifting and volcanism. Over time, the cooling-shrinking crust increases in density and isostatically sinks lower because it is floating on the asthenosphere. Marine sediments gradually blanket the aging oceanic crust as it moves away from the spreading center. The layer of sediment grows thicker and thicker as aging ocean crust moves away from a mid-ocean ridge.
Structure of the Oceanic Lithosphere: Ophiolite Sequences
The character of the rocks and sediments on the seafloor are similar in most locations around the world. These materials appear in a pattern called an ophiolite sequence. Ophiolite sequences can be found exposed in coastal mountain ranges and in ancient mountain systems where portions of the ocean crust have been split away and pushed up by tectonic activity. For instance, ophiolite sequences, or portions of them, are exposed throughout the Coast Ranges of central and northern California.
Four distinct layers of oceanic lithosphere (combined are called an ophiolite sequence) |
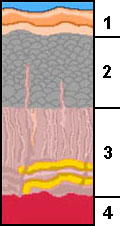 |
• Layer 1: sequence of unconsolidated sediments
Consists mostly of plankton remains (ooze) and fine dust blown in from distance sources including from land (continental deserts sources) and cosmogenous dust. |
• Layer 2: consisting of pillow lavas
Forms from basaltic lava erupting on the surface of the seabed, rapid cooling from exposure to seawater creates the pillow-like structure of the lava beds on the sides of underwater volcanoes. |
• Layer 3: interconnected dikes called sheeted dikes
Newly cooled igneous rock formed at depth shrinks and fractures as it cool, allowing more magma to inject upward into new fractures in the expanding rift zone along the axis of a spreading center |
• Layer 4: gabbro (like basalt but slowly cooled at depth) and serpentinite (seafloor crustal rocks altered by exposure to seawater). |
Features of an Ophiolite Sequence
• Layer 4: represents rocks derived from the upper mantle or rocks formed from the crystallization of magma derived from the mantle. Rocks preserved in Layer 4 deposits are typically a gabbro of ultramafic composition: pyroxenite and peridotite (rocks rich in olivine and other ultramafic minerals) (Figure 15-33). Over time, exposure to seawater and changes in pressure and temperature, the convert to the metamorphic rock, serpentinite.
• Layer 3: Magma injected into parallel fractures above magma chambers along spreading centers create a sheeted dike complex composed of igneous rocks of ultramafic to mafic composition (gabbro and basalt) (Figure 15-34).
• Layer 2: Pillow basalts form from basaltic lava erupting on the seafloor that is flash-cooled by cold seawater into pillow-shaped pods that stack on the seafloor (Figure 15-35).
• Layer 1: When volcanism ends, marine sediments begin to accumulate on top of the pillow basalts.
Thickness of marine sediments on the seafloor
Where new ocean crust is forming along mid-ocean ridges, there is generally very little sediment. The thinnest layers of marine sediments are generally found in deep-ocean basins near mid-ocean ridges. However, as the ocean crusts ages and moves away from the spreading centers, time allows sediments to gradually accumulate on the seafloor. The blanket of sediment grows thicker and more consolidated as the ocean crust ages. Over time, sediments gradually fill in low places on the seafloor, eventually creating a flat seafloor on many of the world's abyssal plains. The sequence of sediments also increase in thickness in regions closer to continental margins.
Aging of oceanic lithosphere results in chemical and physical changes
Once new oceanic lithosphere forms it begins to cool. The lithosphere is very warm relative to the cold ocean water above it. Large quantities of seawater sink into the new ocean crust and chemically reacts with it. These chemical process are a form of metamorphism. Ultramafic rock (rocks enriched in magnesium and iron) that formed deep in the upper mantle and oceanic lithosphere can gradually be altered into serpentinite (Figure 15-36). Large amounts of serpentinite are exposed in the Coast Ranges of northern California where old ocean crust has been pushed up and exposed in the mountain ranges.
|
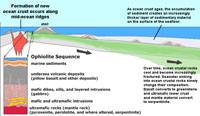
Fig. 15-32. Formation of oceanic lithosphere produces an ophiolite sequence (ocean crust) along spreading centers associated with mid-ocean ridges.
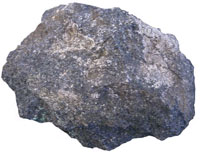
Fig. 15-33. Gabbro (varieties pyroxenite and peridotite) is a dominant dark crystalline igneous rock type formed in oceanic lithosphere.
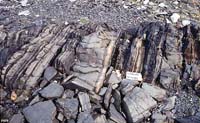
Fig. 15-34. Sheeted dikes form as magma is injected upward into fractures in cooling lithosphere along rifting spreading centers.
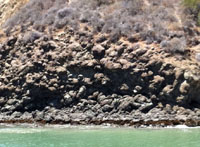
Fig. 15-35. Pillow basalts exposed near Avila Beach, central California.
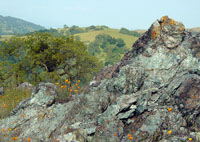
Fig. 15-36. Serpentinite (the State Rock of California) is a metamorphic rock derived from altered oceanic lithosphere. This outcrop is part of a large mountain-sized block of oceanic crust exposed in the mountains near San Jose, California. |
14.19
Formation and Destruction Cycle of Oceanic Lithosphere
The discussion below is a review of the formation and destruction of oceanic lithosphere (discussed in Chapter 6). |
Continental rifting—the birth of a new ocean basin
• A new ocean basin begins with a the formation of a continental rift (example: the African Rift valleys, Figure 15-37).
• The Red Sea is an example of a rift valley that has lengthened and deepened into a narrow linear sea
• If spreading continues the Red Sea will grow wider and develop an oceanic ridge similar to the Atlantic Ocean.
Subduction—the destruction of oceanic lithosphere
• Oceanic lithosphere subducts because its overall density is greater than the warmer underlying mantle. Sinking slabs of ocean lithosphere may actually pull more lithosphere behind it. This may be part of the driving forces responsible plate motion.
• Subduction of older, colder lithosphere results in STEEP descending angles of the sinking oceanic lithosphere.
• Younger, warmer oceanic lithosphere is more buoyant and angles of descent are SHALLOW.
• Research indicates that parts, or even entire oceanic basins, have been destroyed along subduction zones.
Destruction of oceanic lithosphere adds new material to continental crust
As oceanic lithosphere sinks back into the asthenosphere it carries large quantities of seawater and sediment with it. As it sinks, the increased heat and pressure forces water and gases out of the rock. This combination of trapped water and gases allows some of the material melt. The resulting magma that forms is depleted in iron and magnesium, but enriched in aluminum and silica (felsic in composition). This is because mafic minerals have both higher melting temperatures and higher density than felsic minerals.
As a result this natural refining process occurs... denser, mafic material sinks back into the mantle whereas the molten felsic material (along with trapped water and gases) separate and work their way back to the surface. This results in the formation of volcanoes in the region above where subducting lithospheric slabs are sinking. This felsic material is less dense and becomes incorporated into new continental crust. Over long periods of time, enough felsic material accumulates to build continents, a process that may take billions of years. |
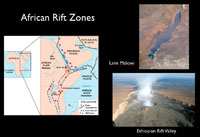
Fig. 15-37. Map and features associated with African continental rift zones.
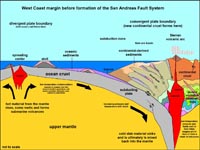
Fig. 15-38. A plate tectonics model illustrating the formation and destruction of oceanic crust and the formation of continental crust. |
14.20
Marine Sediments
Marine sediments (and sedimentary rocks of marine origin) are derived and deposited in different ways than sediments deposited on land. The discussions below focus on the nature of sediments deposited in the marine environment. The discussion below expands on what is described in Chapter 9 (origin of sediments) and Chapter 10 (sedimentary rocks). Marine sediments are generally classified by their origin. |
Sediments deposited in oceanic setting reflect the character of the physical environments where they are deposited. Waves and currents influence sediment deposition in nearshore and shallow- to deep-water setting near land masses. In contrast, there is little current activity influencing sediment deposition in the deep ocean basins far from shore.
Sediments deposited in the marine environment are generally classified into 4 separate groups based on their origin (discussed below):
a) Cosmogenous sediments: material that falls to the Earth surface from outer space.
b) Hydrogenous sediments: material precipitated directly seawater.
c) Lithogenous sediment: material derived from erosion of other rocks, typically from continental sources.
d) Biogenous: material formed from the accumulation of remains of living organism.
|
14.21
Cosmogenous Sediments
Cosmogenous sediments originated from outer space. Scientists have used satellites to estimate how much material enters the Earth's atmosphere. Current estimates from satellite data suggesting about 100 to 300 tons (mostly cosmic dust) hits earth each day. This is just a tiny fraction of the sediments generated on Earth each day. However, early in the history of our Solar System, Earth and other planets, moons, comets and asteroids formed from the gravitational accumulation of extraterrestrial material, but by 4.5 million years ago, most of this cosmogenous accumulation had significantly diminished. However, cosmogenous materials including iron-nickel and stony meteorites can be found. Although a relatively insignificant source of sediment, meteor fireballs disintegrating in the atmosphere contribute dust that can accumulate measurable amounts in parts of some ocean basins.
Extraterrestrial impacts have changed life on Earth repeatedly, including the mass extinction at the end of the Mesozoic Era associated with the extinction of dinosaurs and many other forms of life on land and in the oceans. Tektites are silica glass generated by extraterrestrial impacts—asteroids exploding on the surface and molten material is ejected into the atmosphere where it condenses into a glass-like material.
In deep ocean basin settings, far from shore, deposition of sediments may be very slow. Cosmogenous material, mostly in the form of dust particles, are mixed with dust and fine volcanic ash blown in from distant land sources. These deposits form abyssal clay deposits or are commonly diluted by organic-rich mud materials (oozes, discussed below). |
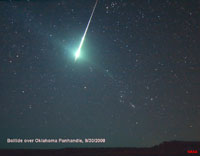
Fig. 15-39. A meteor fireball (a bolide) disintegrates in the night sky over Oklahoma. |
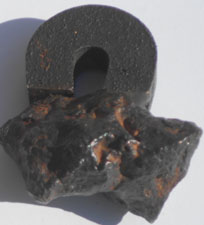
Fig. 15-40. Iron-nickel meteorite from the Diablo Canyon meteor crater area, Arizona (see below) |
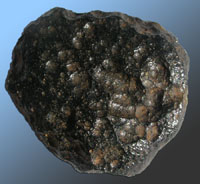
Fig. 15-41. A tektite is a ball of glass-like material ejected by an asteroid impact. |
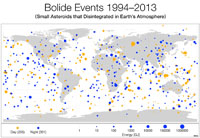
Fig. 15-42. Known locations of bolide events (1994 to 2013). Bolides are meteor fireballs that explode when entering the atmosphere. Few reach the ground or oceans, however they produce large clouds of dust. |
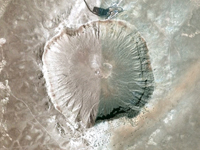
Fig. 15-43. Meteor Crater (Diablo Canyon site) near Flagstaff Arizona is a 50,000 year-old asteroid impact site about a mile in diameter and 550 feet deep. Impact ejecta was spread over a large area around the crater. |
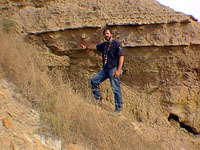
Fig. 15-44. Evidence of the Cretaceous-Tertiary extinction boundary event is preserved in sediments in many locations around the world. This one is in South Dakota. In many places it preserves cosmogenous materials. |
14.22
Hydrogenous Sediments
Hydrogenous sediments are sediments directly precipitated from water. Examples include rocks called evaporites formed by the evaporation of salt bearing water (seawater or briny freshwater). Although salts are a major component of seawater, comparatively little of it gets deposited excepted along the shores of shallow, restricted bays along coastlines and in continental interior basins in arid regions of the world.
Evaporites (Salts)
An evaporite is a rock composed of salt minerals left behind by the evaporation of salty water. Examples include rock salt (NaCl) and gypsum (CaSO4 • x H2O).
Rock salt—a rock dominantly composed of sodium chloride (NaCl - the mineral halite; Figure 15-45). Rock salt is an evaporite formed in restricted basins with an inflow of seawater located in an arid environmental setting.
Gypsum and anhydrite—gypsum is a mineral composed of hydrous calcium sulfate (CaSO4-2H2O); an evaporite mineral used in the manufacture of plaster and fertilizers. Gypsum is deposited by concentrated seawater and by evaporation of freshwater in arid regions. Crystals of gypsum are common in soils in arid regions. If gypsum looses its water content, it is becomes a mineral called anhydrite (Figure 15-46).
Salts are precipitated when sea water (or briny lake water) is concentrated by evaporation (Figures 15-47 and 15-48). Shorelines along the oceans in hot arid regions of the world are places where salt, gypsum and anhydrite are being deposited today. Places where salts (evaporites) are actively accumulation include around the Red Sea and Persian Gulf. Salt deposits are also forming in isolated, internally drained lake basins around the world including the Great Salt Lake in Utah and the Dead Sea.
Iron-Manganese Nodules
Iron-manganese nodules form on the ocean bed (mostly in the deep Pacific) from the slow precipitation of metal oxides in the absence of other kinds of sediments. It may take many millions of years for an individual manganese nodule to grow on the deep seafloor (Figures 15-49 and 15-50). Deposits of them cover the seafloor only in regions located very far away from lithogenous sediment sources.
|
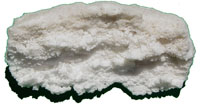
Fig. 15-45. Rock salt is the name for rock composed mostly of the mineral halite. Rock salt precipitates directly from seawater. |
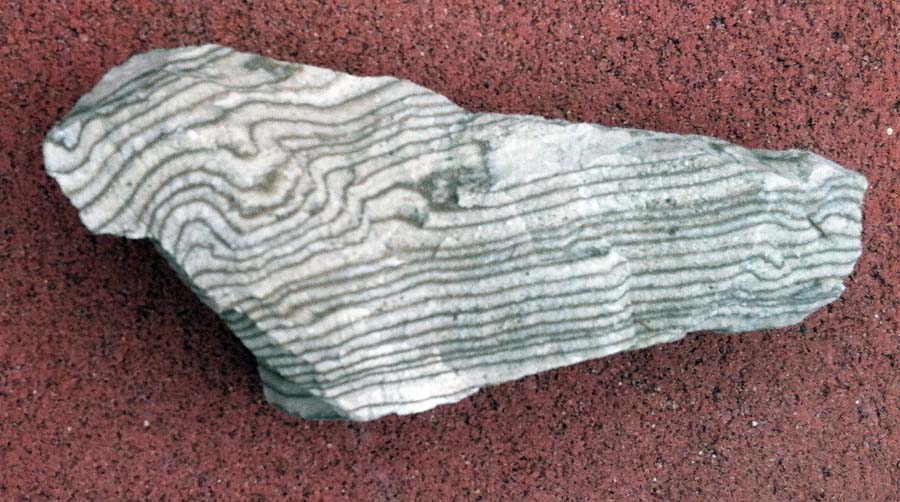
Fig. 15-46. Anhydrite was originally deposited as gypsum with thin layers of organic matter before it looses its water content. |
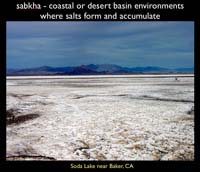
Fig. 15-47. A sabkha is a desert coastal environment is where salts, including halite and gypsum, are commonly deposited. |
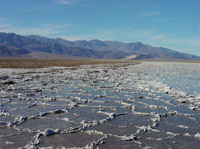
Fig. 15-48. Polygons of salt crystals (halite) being deposited on a salt pan on a dry lake bed in Death Valley National Park, California |
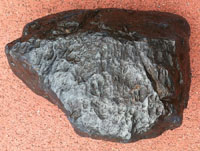
Fig. 15-49. An iron-manganese nodule forms very, very slowly from direct precipitation on the seafloor. |
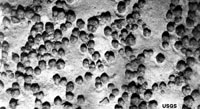
Figure 15-50. Iron-manganese nodules on the deep seafloor near the Puerto Rico Trench. |
14.23
Lithogenous Sediments
Lithogenous sediments form through the processes of weathering and erosion of materials exposed on land and along coastlines. Lithogenous sediments consist of solid fragments of inorganic or organic material that come from the weathering of rock and soil erosion, and are carried and deposited by wind, water, or ice. Volcanoes and coastal mountain ranges are major sources of lithogenous sediments. Volcanic ash clouds from erupting volcanoes and sediments carried by rivers in flood dump large quantities of sediment into ocean basins. In addition, shoreline erosion by wave action is also a major source of lithogenous sediments in some regions. Lithogenous sediments are also commonly called terrigenous sediments because they are derived dominantly from continental sources. They are also called clastic sediments because they are made up of rock fragments derived from other rocks—a clast is a Greek word for a rock fragment (discussed in Chapters 8 and 9).
Lithogenous sediments are:
• Mostly small pieces of broken rock transported to ocean from the land (wind, rivers, glaciers, coastal erosion, etc.)
• Generally form deposits rapidly (such as sand on a beach or a river delta)
• Can form in high energy environments and have coarse grain sizes (coarse sand, gravel, cobbles, and boulders).
• Beach sand is mostly composed of the quartz (SiO2), a mineral which very resistant to weathering.
• Most lithogenous sediments eventually are deposited along the margins of ocean basins.
• Some is deposited into the deep ocean by currents and underwater landslides near continents, and far offshore, lithogenous sediment of fine silt and clays, some as desert dust, forest-fire ash, or volcanic ash blown in by the wind. |
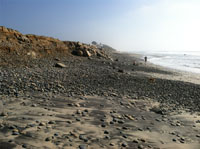
Fig. 15-51. Sand and gravel on SoCal beaches are typical lithogenous sediments. This view is of South Carlsbad State Beach, California. Lithogenous sediments accumulate offshore, building up continental shelves and flooding int deep ocean basins along continental margins. |
14.24
Continental-Derived Lithogenous Sediments
Continents shed vast quantities of sediments into adjacent ocean basins. Rivers in flood transport vast quantities of gravel, sand, silt, and clay (mud) into ocean basins. River deltas are vast accumulations of sediment along the boundary between coastal plains and continental shelve, however the deltas exposed on land are only a small fraction of the material deposited at sea. Most of the sediment is carried to sea by flood waters where they are redistributed offshore by wave and currents, especially during major tropical storms. For example, the Ganges River floods each year as annual monsoonal storms drench to Himalayan Mountains and Indian subcontinent. Flood waters dump vast quantities of sediment into the Bay of Bengal in between India and Bangladesh (Figure 15-52). The influx of sediments supplied by the Ganges River supports a large mangrove forest (which traps some sediment to form new land). However, most of the mud continues to move off shore to contribute to a massive accumulation of sediments, many miles deep, along the outer continental margin beneath the Bay of Bengal.
Volcanoes also contribute large quantities of sediments to ocean basins. Thick accumulations of volcanic sediment build up along the volcanic island chains along the margin of the Pacific Ocean (such as the Andes Mountains of South America, the Aleutian Island Chain, Japan, Philippines, Indonesia, and others). Volcanoes in the western Pacific region are also subject to severe erosion from tropical storms. Heavy rains from tropical storms produce lahars (volcanic mud flows) and floods that move vast quantities of sediment. For example, the volcanic eruptions of Mount Pinatubo in the summer of 1991 released an estimated 8 to 10 cubic kilometers of ash and erupted materials (Figure 15-53). The ash clouds rose to 35,000 feet into the atmosphere and spread ash and sediments both across the landscape and into adjacent Pacific Ocean basin. The eruption occur the same time that Typhoon Yunka struck. Storm flood waters and mudflows during and after the eruption also dumped vast quantities of volcanic material into the sea. |
Fig. 15-52. The Ganges River transports large quantities of sediments derived from the Himalayan Mountains region into the Indian Ocean.
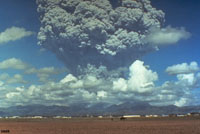
Fig. 15-53. A massive eruption of Mount Pinatubo volcano in the Philippines. |
14.25
Turbidity Currents and Development of Submarine Canyons and Fans
A turbidity flows is a turbid, dense current of sediments in suspension moving along downslope and along the bottom of a ocean or lake. In the ocean, turbidity currents can be massive episodic events. They typically form and flow down through a submarine canyon (carved by previous turbidity flows) and accumulate near the base of the continental slope on deep-sea fans. Turbidity flows produces deposits showing graded bedding (Figure 15-54). Slowing turbid currents drop their coarser fractions first (gravel and sand) and the finer silt and clay fractions settle out last. Sediments deposited by turbidity currents are called turbidites.
A deep-sea fan is a fan- or delta-shaped sedimentary deposit found along the base of the continental slopes, commonly at the mouth of submarine canyons. Deep sea fans form from sediments carried by turbidity flows (density currents) that pour into the deep ocean basin from the continental shelf and slope regions and then gradually settle to form graded beds of sediment on the seafloor. Deep-sea fans can extend for many tens to hundreds of miles away from the base of the continental slope and an coalesce into a broad, gently sloping region called a continental rise.
Graywacke is a fine-to-coarse-grained sedimentary rock consisting of a mix of angular fragments of quartz, feldspar, and mafic minerals set in a muddy base (commonly called a dirty sandstone or mudstone because of its mixed size fractions and brown appearance). Graywacke is the general term applied to sediments deposited by turbidity flows, and they commonly show graded bedding. Graywacke is common in the Coast Ranges of California and other active continental margin regions. It is exposed on land where tectonic forces push up rocks that originally formed in the deep ocean (example in Figure 15-57). Turbidites (deposits associated with turbidity flows) commonly appear as inter-bedded layers of graywacke sandstone and shale. Conglomerate typically occurs in thicker beds and were originally deposited as gravel and mud on ancient submarine fans closer to the mouths of submarine canyons or in channels carved into the seabed. |
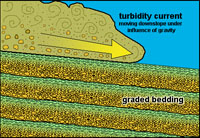
Fig. 15-54. Turbidity flows are essentially underwater landslides or density-driven currents. Sediments laden with sediment are heavier than clear seawater. |
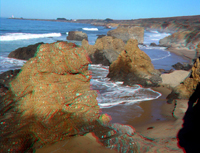
Fig. 15-55. Seas stacks composed of submarine channel deposits (mostly conglomerate) exposed at Gazos Creek State Beach, California |
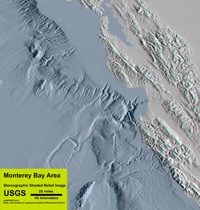
Fig. 15-56. Turbidity currents scour canyons in the deep offshore environment and deposit sediments in the deep ocean. Deep-sea fans build up the continental rise region at the base of the continental slope and spread for hundreds of miles seaward. |
Fig. 15-57. Cretaceous-age turbidites (turbidity current deposits) at Bean Hollow State Beach, California. Each layer represents an undersea storm (turbidity flow) that spread across a deep sea fan on a continental rise. They were pushed up by tectonic uplift along the coast. |
14.26
Abyssal Clays
Abyssal clays are
very fine-grained sediments, mostly clay minerals and iron-rich mineral dust that are blow in by the wind from distant terrestrial sources. Much of the abyssal clay components are derived from dust storms in the world's desert regions and from explosive volcanic eruptions that can blow fine particles high into the atmosphere. Abyssal clays are also fine-grained material carried and redistributed by ocean currents such as tail end of far-turbidity currents that can travel hundreds to even thousands of miles away from continental margins. Abyssal clays in the deep ocean basins accumulate very slowly relative to other ocean sediments. Abyssal clays dominate sediments on the seafloor in the northern Pacific Ocean basin (see discussion on volume and distribution of marine sediments in section 14.37 below). |
14.27
Biogenous Sediments
Biogenous sediments include sediments formed by accumulation of organic materials. Biogenous sediments are mostly composed of the remains of organisms—including skeletal remains of microplankton (both plants and animals), plant remains (algae, wood, roots, and leaves) and remains of larger animals including shells of invertebrates, such as shells, coral fragments, and fish and other vertebrate teeth, bone, and scales, and fecal material left behind by any type of organism. Biogenous sediments can form in both terrestrial and marine environments. Biogenous sediments may be partly mixed with lithogenous sediments (continental-derived sediments) in coastal regions, particularly where streams and rivers contribute sediments. For instance, quartz beach sand is not biogenous, but ground up an worn fragments of shells is biogenous. Some beach sands consist entirely of shell and coral fragments.
Biogenous Sediments In the Marine Environment
Bioaccumulation is the buildup of organic remains, such as deposits associated with coral reefs, shell or bone beds, and algae and ooze (calcareous and siliceous). On land bioaccumulation in swampy environments produces peat beds (with burial and time, peat eventually can be converted to coal). In many passive margin regions in tropical regions, carbonate sediments form and accumulate forming massive deposits along continental margins.
Carbonate Reefs (or Coral Reefs)
A reef is a general name for a ridge of jagged rock, coral, or sand just above or below the surface of the sea. A carbonate reef is one that is made of skeletal material composed of coral, coralline algae, and other carbonate skeletal material. Carbonate reefs are commonly called coral reefs, but not all organisms that look like corals are actually corals—other organisms that create solid structure (branching or not) include coralline algae, bryozoans, sponges, stromatoporoids, and many other types of invertebrates). Figure 15-58 illustrates the variety of settings and features associated with carbonate depositional environments.
Carbonate (coral) reefs form in clear shallow, warm, tropical marine waters.
Over time, lime sediments are produced by biological activity in and around carbonate reefs. Carbonate reefs grow at rates of 10-30 feet per thousand years. Wave action and currents will erode and redistribute lime sediments offshore where they may accumulate. Over time (millions of years) calcareous sediments build up, creating massive carbonate platforms (becoming regions underlain by limestone that may become strata many thousands of feet thick). Examples of carbonate platform regions include the Bahamas, South Florida, and the Yucatan Peninsula (Figure 15-59 and 15-60).
The world's largest reef system is the reef tracts, islands, and tidal shoals associated with the Great Barrier Reef located along the east coast of Australia (Figure 15-61). The Great Barrier Reef is composed of over 2,900 individual carbonate reefs and about 900 islands stretching for over 1400 miles (2,300 km) along the northeast coast of Australia and encompassing about 133,000 square miles (344,400 km2). It is the largest feature of biological origin on Earth. Similar reef tracts have formed throughout geologic history in other locations around the world.
|
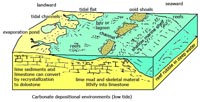
Fig. 15-58. Calcium carbonate depositional environments include coral reefs, keys, shoals, tidal flats, bays, and other coastal and offshore features. |
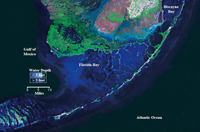
Fig. 15-59. South Florida is part of a growing carbonate platform with the Keys consisting of both ancient and modern reefs, forming a barrier reef complex. |
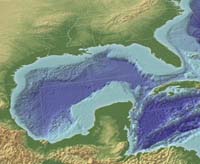
Fig. 15-60. Massive carbonate platforms (how in blue gray) surround much of the Gulf of Mexico. They include continental shelf regions around the Yucatan Peninsula, South Florida, and islands of the Caribbean where biogenous sediments form and accumulate over time. |
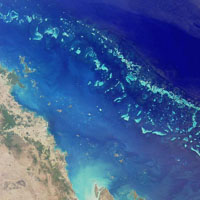
Fig. 15-61. The Great Barrier Reef is the world's largest organic deposit (light blue are keys). The growth of the great reef tract has kept pace with the global rise in sea level since the end of the last (Wisconsin) ice age. |
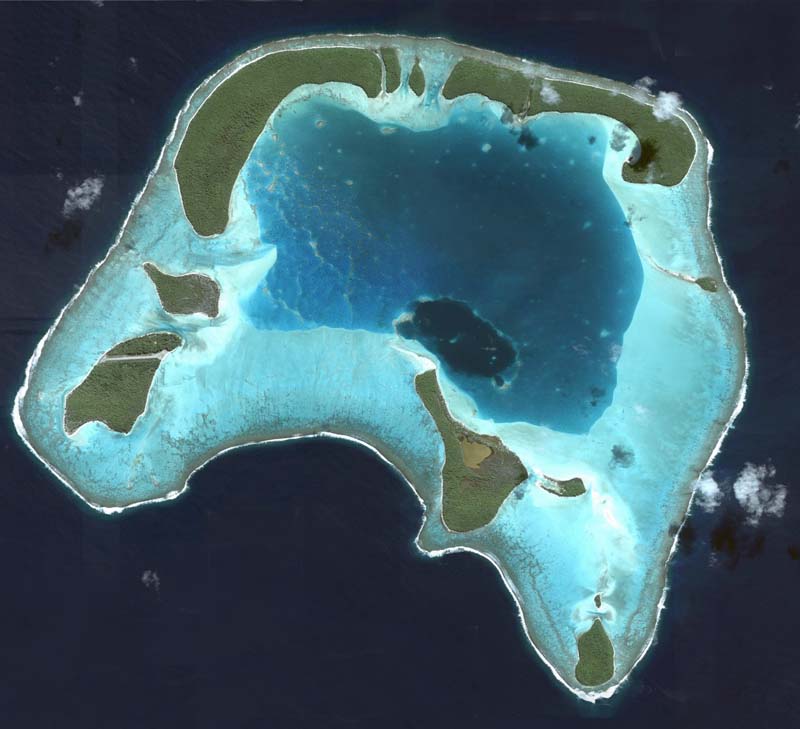
Fig. 15-62. Atolls are volcanic islands or seamounts covered or surrounded by fringing carbonate reefs that build up even long after the volcano stopped erupting. |
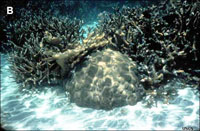
Fig. 15-63. Lime mud, lime sand and larger skeletal fragments accumulate around living coral reefs. Lime sediments are created mostly by organisms feeding on other reef organisms. Storms can erode reefs and redistribute carbonate sediments. |
14.28
Marine Ooze
The oceans are full of many varieties of microscopic organisms, but only several varieties are responsible for generating vast quantities of biogenous sediments.
Ooze is slimy mud sediment (soft and mushy) on the bottom of an ocean or lake bed formed from the accumulation of skeletal and organic remains of microscopic organisms: phytoplankton (plants) and zooplankton (animals).
• Oozes can be dominantly calcareous or siliceous in composition.
• To be considered an ooze sediment must consist of grater than 30% biogenous material (Figure 15-64).
• Oozes form slowly - accumulating at a rate of 1/2 to 2 1/2 inch per 1000 yrs.
• Oozes form in low energy environments and are very fine grained (clay sized particles).
|
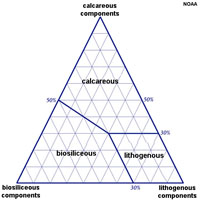
Fig. 15-64. Components of oozes: calcareous, biosiliceous, and lithogenous materials |
14.29
Calcareous Oozes
Calcareous oozes are sediments dominantly composed dominantly of calcium carbonate (CaCO3). Two dominant groups of microorganisms that contribute carbonate remains: Coccolithopores (phytoplankton) and Foraminifera (zooplankton)
|
14.30
Coccolithopores
Coccolithopores are single-celled marine phytoplankton (microscopic plants) that live in large numbers throughout the upper layers of the ocean. Unlike any other plant in the ocean, coccolithopores secrete shells of microscopic plates made of calcite (CaCO3). These scales, known as coccoliths, are shaped like hubcaps and are only three one-thousandths of a millimeter in diameter (Figure 15-65). Coccolithopores are part of base of the food chain and contribute vast quantities of coccoliths as sediment to large regions of the ocean basins. Coccoliths are concentrated in calcareous ooze.
Coccoliths first appear in the fossil record in Triassic time. Because they are composed of low-magnesium calcite (the most stable form) they are easily fossilized and preserved in sedimentary rocks.
What is a Coccolithopores? (NASA)
|
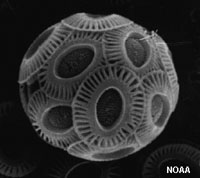
Fig. 15-65. A Coccolithopores is covered with calcareous plates called coccoliths. |
14.31
Foraminifera (Forams)
Foraminifera (or forams) are a large group of single-celled zooplankton, most species have calcareous shells (or tests). Their shells are commonly divided into chambers which are added during growth and form patterns including spirals, open tubes, or hollow spheres (Figure 15-66). Depending on the species, the shell may be made of crystalline calcite, organic compounds, or sand grains and other particles cemented together. They are usually less than 1 mm in size, but some species grow much larger, reaching up to 20 cm. The majority of foraminifera species are benthic (meaning they live on or within the seamounts sediment) while typically smaller varieties are floaters (planktonic) in the water column at various depths. Foraminifera are found in all depths of the ocean, although deep ocean varieties do not have calcareous tests. They contribute a significant volume of sediments to carbonate reefs and a major component of carbonate oozes throughout ocean basins.
Over 10,000 species of foraminifera are recognized, both living and fossil. They first appeared in the fossil record in Cambrian time (over 500 million years ago. |
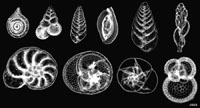
Fig. 15-66. Examples of foraminifera tests.
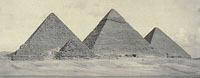
Fig. 15-67. Pyramids of Giza, Egypt are constructed with foraminifera-rich limestone. |
14.32
Chalk
Chalk is a soft, fine-grained, white to grayish variety of limestone that is composed of the calcareous skeletal remains of microscopic marine organisms including coccoliths and foraminifera. Some of the purest varieties can have up to 99 percent calcium carbonate. The White Cliffs of Dover, England are one of the most iconic landscape features in the United Kingdom (Figure 15-68). The White Cliffs consist of Cretaceous-age chalk deposited about 89 to 85 million years ago in more tropical conditions than exist in the region today. The layers of chalk reach nearly 500 meter thick. The sediment the chalk formed from was coccolithopore ooze. |
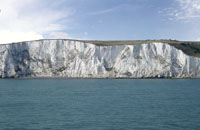
Fig. 15-68. The White Cliffs of Dover, England are chalk. |
14.33
Siliceous Oozes
Siliceous oozes are sediments dominantly composed dominantly of SiO2 (silica).
Two dominant groups of organisms that contribute siliceous remains: diatoms and radiolarians.
Diatoms
Diatoms are the most common plankton. Diatoms are phytoplankton (single-celled microscopic marine plants) (Figure 15-69).
• Diatoms are most common in polar regions, but are also know from tropical and subtropical regions as well.
• Very important for upwelling nutrients (where deep water rich in .
• Diatoms have many economic uses including in beer filters, pool filters, and optical glass. |
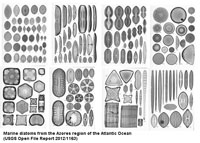
Fig. 15-69. Example of diatoms. These are images taken with a microscope. |
14.34
Radiolarians
A radiolarian is a single-celled aquatic animal (zooplankton) that has a spherical, amoeba-like body with a rigid spiny skeleton of silica. There are hundreds of known species of radiolarians (See a list on radiolaria.org website).
Figure 15-70 is a photomicrograph depicting the siliceous tests of ten species of marine radiolarians.
Upon death, their tests can accumulate on the seamounts and form siliceous marine sediments known as radiolarian ooze (a form of siliceous ooze). Radiolarians first appear in the geologic record in early Cambrian time and have experienced several periods of proliferation and extinctions as recorded in the geologic record.
Today,
radiolarians are more common in equatorial regions.
|
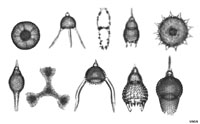
Fig. 15-70. Example of Radiolarian skeletons (tests). These are images taken with a microscope. |
14.35
Chert
Chert is a fine-grained siliceous sedimentary rock. It is a hard, dense, and consist chiefly of interlocking microscopic crystals of quartz and may contain opal. It has a conchoidal fracture and may occur in a variety of colors. Most chert forms from compaction and recrystallization of siliceous microplankton remains—siliceous ooze eventually looses its water content and recrystallizes, and turns into layers of chert (Figure 15-71).
Organic residues preserved as chert beds are known from rocks dating back to early Precambrian time. Banded-iron formations (BIFs) are composed of inter-bedded layers of iron-oxide minerals and chert, and are thought to be biogenous in origin (Figure 15-72). Younger marine cherts are mostly formed from diatoms and radiolarian oozes. |
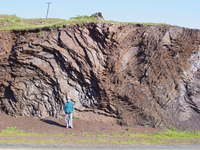
Fig. 15-71. Layers of marine chert exposed in the Marin Headlands, California |
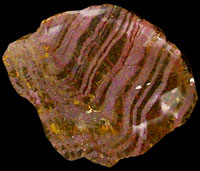
Fig. 15-72. Banded-iron formation (with chert) from the Precambrian era. |
14.36
Neritic and Pelagic Sedimentary Deposits
The term neritic is used to described the shallow part of the ocean near a coast and overlying the continental shelf.
Neritic sediments are generally shallow ocean deposits formed close to land. They are dominated by lithogenous sources and are typically deposited quickly. Neritic sediments are moved and sorted by ocean waves and currents. Coarser-grained materials are deposited nearshore or near the mouths of submarine canyons. Finer materials are commonly winnowed out and carried away to quieter-water settings. Carbonate Reefs (also called coral reefs) form in warm, shallow, and clear tropical waters in locations where they are mostly isolated from sources of sediment eroded from land.
Neritic sediments cover about ¼ of seafloor and are near landmasses.
The term pelagic means of or relating to the open sea, particularly the upper layers of the ocean.
Pelagic sediments are generally deep-water deposits mostly oozes (see below) and windblown clays—abyssal clays deposited far away from continental sources of sediments. They are typically very-fine-grained sediments that are deposited slowly. Because they are deposited far beyond the continental margins they are typically less lithogenous and more biogenous depending on biologic productivity.
Pelagic sediments cover about ¾ of seafloor and are mostly in deep water.
The distribution of neritic or pelagic sediments is controlled by proximity to sources of lithogenous sediments (i.e.: landmasses) and the productivity of microscopic marine organisms or shallow water reef organisms.
|
14.37
Volume and Distribution Of Marine Sediments
Of the 4 types of sediments, lithogenous and biogenous sediments are the most abundant on Earth today (Figures 15-73 and 15-74). Lithogenous sediment dominate the regions adjacent to continental landmasses (continental margins). The lithogenous sediment accumulations along continental margins can be many miles thick, especially where rivers have dumped large quantities of sediments for long periods of geologic time. Biogenous sediments accumulations can also be massive, particularly in locations where warn, shallow seas allow massive reef tracts to persist for long periods of time, such as with the Australian Great Barrier reef. Planktonic remains blanket the seamounts in large regions of the world's oceans. In contrast, cosmogenous and hydrogenous sediments are generally insignificant in comparison, but have important scientific and economic significance where they occur.
Sedimentary rocks of marine origin are also exposed throughout the world's continents, covering about half of the exposed land on the surface. This sedimentary cover blanketing continental areas was originally deposited mostly in coastal environments, in shallow seas flooding shallow continental basins, on continental shelves and in ocean basins along the margins of continents (Figure 15-75). Most of these sedimentary rocks that blanket much of the continents formed in the last several hundred million years. Even more massive quantities of sediments occur along continental margins in ocean basins. In many places around the world the thickness of sediments eroded from continental landmasses and volcanic chains and deposited in the adjacent ocean basin can be many miles thick! The thickest volume of sediments typically underlie the outer margins of continental shelves, especially on passive continental margins (Figure 15-76). Sediments are thinnest or nonexistent on new ocean crust forming along mid-ocean ridges.
Most lithogenous sediments are on or near a landmass
• Coarser sediments accumulate closer to shore,
• Finer sediments are winnowed by waves and currents and are transported farther from shore to quieter water settings where they can settle out. |
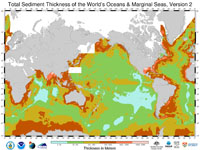
Fig. 15-73. Thickness of sedimentary deposits along continental margins.
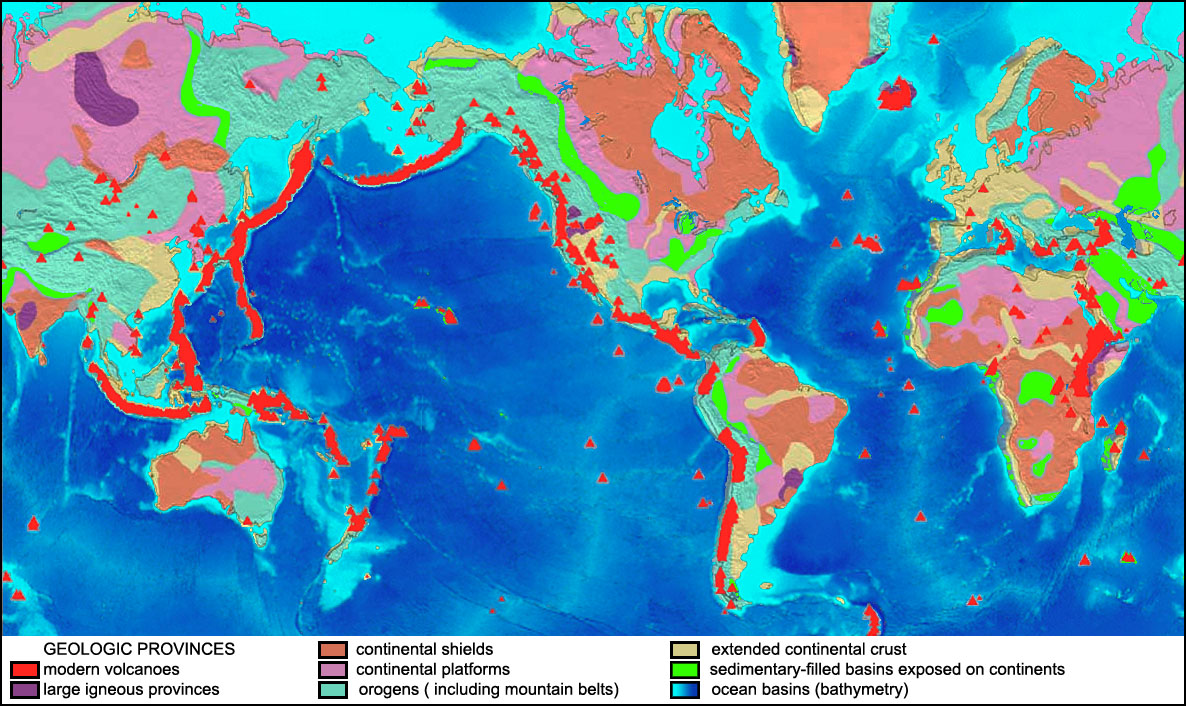
Fig. 15-75. Map of geologic provinces of the world. Sediments and sedimentary rocks not only cover much of the world's seabed but also cover large regions of the continents that were once under water. |
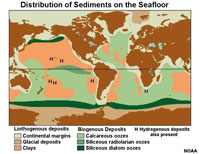
Fig. 15-74. Distribution of sediments on the seamounts by type.
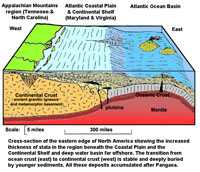
Fig. 15-76. Continental margins are places where large quantities of lithogenous and biogenous sediments accumulate. They are thinnest or missing on new ocean crust forming on mid-ocean ridges. |
|