Chapter 11 - Metamorphic Rocks & Processes |
|
11.1
Metamorphosis literally means a change in form. Metamorphism refers to changes in rocks—it is the mineralogical, chemical, and structural adjustment of solid rocks to physical and chemical conditions imposed at depth below the surface. Metamorphism typically occurs below surficial zones where processes of sedimentation, compaction, and cementation take place. Metamorphism may take place where a rock is subjected to conditions unlike those in which it originally formed. A metamorphic rock is a rock that was once one form of rock but has changed to another under the influence of heat, pressure, or fluids without passing through a liquid phase (without completely melting).
This chapter describes and follows the changes that happen to rocks as they are buried deeper in the Earth, either by isostasy or forces associated with mountain building in a variety of tectonic settings. Rocks go through metamorphic changes whenever they go through changes in heat, pressure, and exposure to chemically-reactive fluids—involving a variety of gazes and liquids (mostly water and steam) that exist under pressure within the Earth. |
Click on images for a larger view throughout this website. |
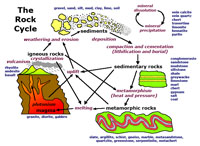 |
Fig. 11-1. The Rock Cycle includes metamorphic rocks and processes.
|
11.2
Origins of Metamorphic Rocks
Metamorphic rocks form where a pre-existing rock is subjected to conditions unlike those in which it formed. Metamorphic rocks are not melted as they form (that would be igneous); the mineralogical change takes place in a solid state. Metamorphism and metamorphic rocks are part of the rock cycle (Figure 11-1). Most metamorphic rocks are made up of common rock forming minerals but may also contain minerals that only form under metamorphic conditions (of which there are an interesting variety). Many different processes can form metamorphic rocks. Both sedimentary and igneous rocks can become metamorphic rocks. Igneous activity can also cause metamorphism as rocks are heated or even partially melt, releasing water and gases and some of their low-temperature melting minerals (such as quartz and micas).
Metamorphism takes place as rocks sink deeper into the Earth's crust as they are exposed to increasing degrees of pressure and temperature. The term, geothermal gradient, refers to the increase in temperature with increasing depth in a area or region. On average, the geothermal gradient is about 1° Fahrenheit per 70 feet (or about 25 °C per kilometer), but may be higher in regions along plate boundaries, mountain belts, or around regions of volcanism and hotspots in the crust. Many minerals that are stable at earth-surface conditions become unstable and change both physically and chemically under increasing pressure and temperature conditions, forming new minerals or reorienting crystal structures. The opposite may occur as rocks and minerals that are stable deep in the crust may undergo retrograde metamorphism as they rise and are exposed to lower pressures and temperatures closer to the surface.
|
11.3
Important Metamorphic Minerals illustrated
Many common rock-forming minerals (including silicate and non-silicate minerals) found in intrusive igneous and sedimentary rocks can occur in metamorphic rocks including quartz, feldspars, micas (biotite and muscovite), amphibole, pyroxene, calcite, dolomite, and iron-oxide minerals (Figure 11-2). Metamorphic processes can cause new minerals to form (often at the expense of others). However, some minerals are unique to metamorphic settings, including the following (illustrated in Figure 11-3).
Talc is a fine-grained white, greenish, or gray mineral, Mg3Si4O10(OH)2, having a soft soapy feel (#1 on the Mohs Hardness Scale).
Chlorite is a dark green mineral consisting of a basic hydrated aluminosilicate of magnesium and iron, often formed by metamorphic alteration (low grade or retrograde greenschist metamorphic facies).
Epidote is a yellow-green crystalline mineral, common in metamorphic rocks, consisting of a hydroxyl silicate of calcium, aluminum, and iron.
Enstatite is greenish-to-brown translucent magnesium-silicate mineral (a member of the pyroxene group) that occurs in metamorphic and igneous rocks, as well as stony meteorite.
Kyanite is a blue or blue green aluminum-silicate mineral that forms in bladed crystal masses; used in the manufacture of heat-resistant ceramics.
Serpentine is a common name used for any of a group of greenish, brownish, or spotted hydrated magnesium silicate minerals [such as Mg3Si2O5(OH)4 ] that is mined as a source of magnesium and asbestos
Crysotile is a white to green, fibrous mineral that is a common variety of minerals collective called asbestos. Asbestos minerals have been used for manufacturing and construction that are now banned or restricted from usage because of health-related hazards.
Actinolite is a green mineral of the amphibole group of minerals with bladed or platy crystals; calcium magnesium iron silicate. Actinolite and its related mineral form, tremolite, can have fibrous form that is regulated like asbestos.
Graphite is a carbon mineral that is dark gray to black with a metallic luster and greasy feel; it is used for pencil leads, a lubricant, and for many industrial purposes.
Garnet is most commonly a deep red vitreous silicate mineral found in high-grade metamorphic rocks, sometimes found in gemstone quality crystals. There are actually many varieties of garnet (discussed below).
Staurolite is a dark glassy opaque mineral that in gemstone variety can occur as hexagonal prisms that often consist twinned crystals shaped in the form of a cross.
Corundum is an extremely hard aluminum-oxide mineral (#9 on the Mohs Hardness Scale). Ruby and sapphire are gem varieties of corundum. In manufacturing it is used as an abrasive.
|
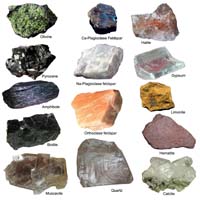 |
Fig. 11-2. All common rock-forming minerals can form or be altered to form other minerals by metamorphism. |
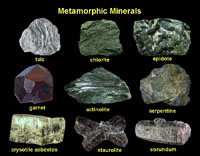 |
Fig. 11-3. Common and important metamorphic minerals (some are gems) |
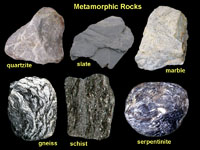 |
Fig. 11-4. Common metamorphic rocks and the related protoliths (sediments or rocks from which they formed). |
11.4
Common Metamorphic Rocks and How They Form
Metamorphic rocks form in stages as rocks undergo increasing metamorphism. Processes involved in metamorphism can completely alter the mineral content and texture of the rocks from which is was derived from (see Figures 11-4 to 11-10). When any pre-existing rock (called a protolith) is heated fluids already within the rock may also be involved in changing the rock to a new form (a metamorphic rock). The word "protolith" is a pneumonic term subjugated from proto meaning first, and lith meaning rock, thus the original rock that was metamorphosed. Different minerals will develop from different original rocks. This is important for looking for gems. Below are descriptions of the most common metamorphic rocks with their protoliths.
Slate is a fine-grained gray, green, or bluish metamorphic rock easily split into smooth, flat plates along cleavage planes. Slate is used for roof shingles and black boards. Slate displays slaty rock cleavage (see below).
Phyllite is a fine-grained metamorphic rock with a well-developed laminar structure, intermediate between slate and schist, very fine micaceous mineral crystals sparkle on surfaces.
Schist is any medium-grained to coarse-grained metamorphic rocks composed of laminated, often flaky parallel layers of chiefly micaceous minerals (biotite, muscovite, chlorite, talc, and others).
Gneiss is a metamorphic rock with a banded or foliated structure, typically coarse-grained (crystalline) and consisting mainly of feldspar, quartz, mica, and may have mafic minerals.
Greenstone is a metamorphic rock derived from any basic igneous rocks (typically altered basalt) colored green by the minerals chlorite, hornblende, or epidote.
Quartzite is a very hard rock with typically a sugary-uniform texture formed from the metamorphism of quartz sandstone or quartz-rich volcanic ash.
Metasandstone is sandstone that has undergone partial metamorphism, but retains enough characteristics to show that it was derived from sandstone.
Metaconglomerate is conglomerate that has been partially metamorphosed, retaining some of the distinct character of original gravel clasts, although they may be stretched or recrystallized.
Metachert is an extremely hard, often brecciated quartz-rich rock that has microcrystalline texture. It can form from the recrystallization of sedimentary chert or silica-rich fine-grained volcanic rocks.
Marble is a crystalline metamorphic rock composed primarily of calcium carbonate (CaCO3). A product of metamorphism of limestone or dolostone. However, high-grade metamorphism typically breaks down and destroys carbonate minerals.
Serpentinite is a metamorphic rock consisting almost entirely of "serpentine group minerals." Serpentinite forms from the metamorphic alteration of ultramafic silicate rocks, particularly peridotite and pyroxenite, where serpentine minerals replace igneous minerals of olivine and pyroxene. Serpentinite is typically a blue, green, gray, or black rock consisting almost wholly of serpentine-group minerals including antigorite, lizardite, brownite, chrysotile, with accessory minerals of chlorite, magnetite, and talc. Serpentinite is the State rock of California and crops out throughout large portions of the northern California Coast Ranges (Figures 11-11 and 11-12).
|
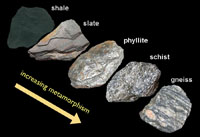 |
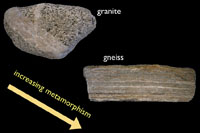 |
Fig. 11-5. With compaction clay-rich mud becomes shale, then with increasing metamorphism changes to slate, phyllite, then schist, and eventually gneiss. |
Fig. 11-6. Granitic rocks can be metamorphosed to gneiss. However, gneiss can ultimately form from many other materials exposed to extreme metamorphism. |
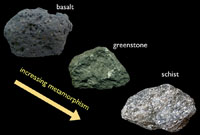 |
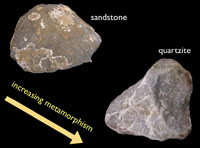 |
Fig. 11-7. Basalt, with increasing metamorphism, turns to greenstone and eventually schist or gneiss. |
Fig. 11-8. When subjected to metamorphism sandstone or quartz-rich volcanic ash becomes quartzite. |
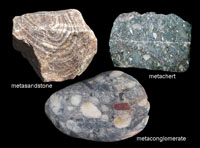 |
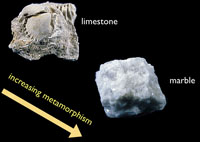 |
Fig. 11-9. Metasandstone, metachert, and metaconglomerate. |
Fig. 11-10. When limestone or dolostone is metamorphosed it becomes marble. |
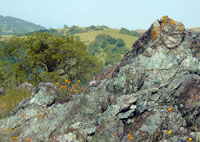 |
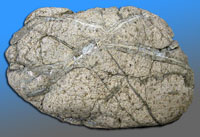 |
Fig. 11-11. Serpentinite is a rock composed mostly of serpentine minerals. Outcrops like this one are common throughout the Coast Ranges of northern California. |
Figure 11-12. Serpentinite showing mineral-filled expansion cracks formed as serpentine minerals replaced the ultramafic protolith (peridotite) derived from the mantle. |
11.5
Processes That Form Metamorphic Rocks
Metamorphism occurs where a rock is subjected to conditions unlike those in which it formed. Metamorphism involves physical and chemical changes that alter pre-existing earth materials (minerals, rocks, sediments). Metamorphism can occur when materials are exposed to heat, pressure, or exposure to chemically active fluids (Figure 11-13). Metamorphism can take place very slowly (such as where rocks very slowly descend in a subduction zone) or very rapidly (such as in the catastrophic effects of an asteroid impact). Heat and pressure are forms of energy. Heat and pressure are the main agents of metamorphic change, but typically only one, either heat or pressure, dominates.
Recrystallization is a metamorphic process that occurs under situations of intense temperature and pressure where grains, atoms or molecules of a rock or mineral are packed closer together, creating a new crystal structure. Despite the physical changes to the rock and minerals, the basic chemical composition remains the same (Figure 11-14).
Heat contributes to the metamorphic processes in two ways (Figure 11-15). First, atoms may combine differently at different temperatures. This means that a mineral stable at one temperature might become unstable at a higher (or lower) temperature and can be converted to a different mineral with a more stable atomic structure. Second, heat makes practically all chemical reactions go faster, meaning that mineral transformations are much easier at higher temperature.
Pressure can control which minerals or forms of minerals are stable. Some minerals may be converted to minerals with similar composition but different atomic packing simply because pressure is increased. Two types of pressure include: 1) confining pressure (pressure on any point is equal in all directions, similar to a balloon held under water), and 2) directed pressure (squeezing of rocks, such as caused by converging tectonic plates)(Figure 11-16).
Chemically active fluids can cause or serve to speed up metamorphic processes (Figure 11-17). Chemical reactions require water, and most proceed much faster as the amount of water goes up. Dissolved ions in the fluid also make those mineral transformations that require chemical changes in the minerals to occur, whether by supplying needed ions or flushing away excess ones. Ongoing compaction forces fluids to migrate out of pore spaces. Because chemically active fluids are also involved in the formation of many igneous and sedimentary rocks and minerals it is sometime difficult to distinguish whether the term "metamorphic" only applies. |
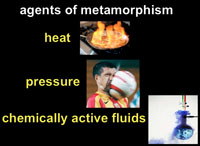
Fig. 11-13. Agents of metamorphism: heat, pressure, and chemically active fluids. |
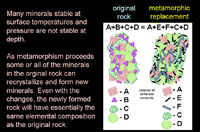
Fig. 11-14. Recrystallization results in the formation of metamorphic minerals and rocks. |
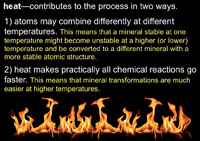
Fig. 11-15. Heat can cause metamorphism by driving chemical reactions. |
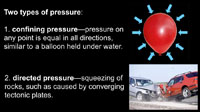
Fig. 11-16. Two types of pressure associate with metamorphism: confining pressure and directed pressure |
Fig. 11-17. Chemically active fluids (dissolved gases in hot water) within rocks can cause metamorphic changes near or deep below the surface.
|
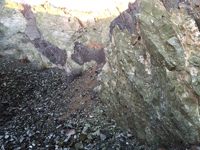
Fig. 11-18. Green epidote in a skarn deposit created by hot fluids altering limestone deposits around a mafic intrusive dike. |
11.6
Metamorphism and Geologic Time
Whereas compaction and cementation are considered processes that convert sediments into sedimentary rocks, these same slow processes are partly responsible for the formation of metamorphic rocks, although under much more extreme conditions. For instance, sand and mud deposited in a river delta environment may, over time, become compacted with increasing burial and cemented by groundwater to become the sedimentary rocks, sandstone and mudstone. However, as sedimentary deposit are buried deeper and deeper into a subsiding sedimentary basin, pressure and temperatures increase to the point that mineral recrystallization will irreversibly take place if conditions are right, producing metamorphic minerals and rock textures associated with metamorphism. The deeper the burial, the greater the changes that occur. For instance, sediments that were once deposited in delta environments along the coastline of Mississippi and Louisiana about 100 million years ago are now buried nearly 7 to 9 miles below the surface and are under the influence of great pressure and heat (almost to the point of melting). One day in the far-distant future, these rocks may be uplifted and exposed at the surface, such as rocks of similar origin now exposed in mountain ranges around the world.
Where Are Metamorphic Rocks Exposed Today?
Most metamorphic rocks consist of materials that have experienced long journeys through geologic time. Metamorphic rocks are mostly exposed in mountain ranges and in old, stable portions of continents that were once the core of mountain ranges long ago. Rocks exposed in the core mountain ranges may have once been buried tens of miles below the surface where heat and pressure changed them from what they had been previously—such as sediments deposited on the seafloor or material ejected from volcanic eruptions.
Note that near the surface rocks are relatively cold and brittle, and will tend to shatter (causing earthquakes), whereas deep in the earth's crust, rocks will tend to bend and flow rather than break when subjected to tectonic motion. The transition between where rocks tend to break rather than flow in a ductile manner is in most places in depths in the range of 8 to 11 miles (13-17 kilometers) and where rocks are in typically in the range of 480° to 750°
Fahrenheit (250° to 400°
Celsius)(Figure 11-19). |
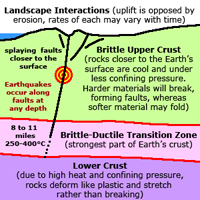
Fig. 11-19. The britte-ductile transition zone in the Earth is at a depth where intense metamorphism takes place because rocks will deform and flow rather than fracture.
|
11.7
Regional Metamorphism
The term regional metamorphism applies to rocks that have largely been changed by pressure. Where metamorphic rocks of this character exist they typically are abundant and distributed throughout a wide geographic area. The name “regional” applies to a large area like a mountain belt (such as the Himalayas, Alps, Appalachians, or Andes). Metamorphic rocks in these regions have been highly compressed prior to being uplifted and exposed by erosion. Rocks associated with regional metamorphism are usually found in exposed mountainous regions or in the shield region of continents where rocks associated with ancient mountain ranges are exposed. Regional metamorphism is most associated with convergent plate boundaries (past and present), and particularly between colliding continental landmasses (Figure 11-20). Continental collisions causes metamorphism to take place on a regional scale. In general, the deeper a rock moves into the crust, the greater degree of metamorphism it endures. As mountain ranges are pushed up, large volumes of rock over a regional area are uplifted and slowly exposed as erosion strips away miles or even tens of miles of rock. The "oldest and deepest" rocks are often exposed in the exposed cores of mountain ranges. |
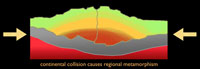
Fig. 11-20. Regional metamorphism is associated with continental collision and crustal shortening. |
11.8
The Manhattan Schist, a famous example of regional metamorphism
Mica schist sometimes contains garnet and several other gem minerals. Mica schist may have started its "geologic journey" as an extremely fine grained rock called clay shale. As the shale was crushed under enormous pressure during metamorphic processes changes and also became hot due to friction and deeper burial in a the crust beneath a mountain range, the clay minerals (which were microscopic grains of mineral) began to grow and enlarge and replaced with the thin sheets mica minerals such as biotite and muscovite. These minerals as we have seen form thin sheets. The Manhattan Schist in the New York City region is an example. Gem garnets, tourmaline, and chrysoberyl has been found in Manhattan Schist.
Figure 11-21 is a highly schematic and old fashion map showing the metamorphic rocks under New York City. The Manhattan Schist, Fordham Gneiss, and Inwood Limestone (actually a marble) are illustrated. These formed in the roots of an ancient mountain chain that rose around 400 million years ago. The oldest rock, the Fordham Gneiss, may be 1.1 billion years old! (Illustration modified from Lobeck, 1932.) |
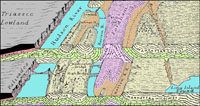
Fig. 11-21. Geologic map of northern end of the New York City area. The Manhattan Schist is the bedrock beneath most of Manhattan Island in New York City. |
11.9
Contact Metamorphism
Some rocks are mainly changed by heat associated with nearby igneous activity. As magma is injected upward or moves along fractures or natural zones of weakness between rock layers, the hot, molten material “bakes” the surrounding rocks to accomplish the change. An analogy would be a piece of white bread being heated and changed to toast. Bread is soft and malleable, but toast is hard and brittle. Toast required the addition of heat energy to make the change. That is the case with rocks too, heat energy and possibly release reactive fluids cause contact changes around an igneous intrusion. Away from the intrusion, the effects gradually reduce until the rock is unheated and in its original condition. The zone of "baking" around an igneous intrusion or beneath a lava flow is called "contact metamorphism." Figure 11-22 shows a contact metamorphic zone around a magma or plutonic rock in a volcanic terrain. The red zone is magma or later plutonic rock on cooling. The zone around it is the aureole or skarn caused by the “ burning” of the surrounding rock. The illustration shows fold rocks that were probably metamorphosed by convergence (regional metamorphism) before the intrusion that then caused contact metamorphosed the surrounding rocks. Igneous intrusions into limestone terranes bake the rocks into marble, and if high-enough temperatures persist, the carbonate minerals are destroyed (CO2 is driven off) and the remain material consists of calcium-silicate minerals. Skarns are often have interesting minerals such as garnets. In addition to the changes cause by heat, hot dissolved-mineral-rich fluids (water, steam and other gases under pressure) introduce other elements (often rare or unusual metals) into the surrounding zone of contact metamorphism.
Figure 11-23 shows a famous example of contact metamorphism along the sides of an igneous intrusion exposed in cliffs of limestone. The Purcell Sill is composed of an igneous intrusive rock (diorite) and cuts through older Precambrian-age sedimentary rocks. This exposure is in Glacier National Park, Montana. Contact metamorphism occurred both on the top and bottom of the sill. Though contact metamorphism is distinct from regional metamorphism that is mainly due to pressure. The igneous rocks that cause the change may be formed by plate tectonics process that also cause regional metamorphism. Usually the area that is contact metamorphosed is much smaller than the area affected by regional metamorphism. |
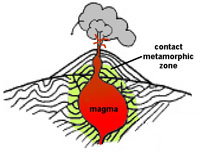
Fig. 11-22. Zones of contact metamorphism occur around igneous intrusions.
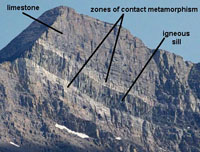
Fig. 11-23. The Purcell Sill displays contact metamorphism in Glacier National Park, Montana. |
11.10
Hydrothermal Metamorphism
Some rocks are mainly changed by hot water and we say these rocks have been changed by hydrothermal metamorphism (hydrothermal implies hot water, Figure 11-24). These are usually around igneous rocks as well, and some people consider it rather different than the other types of metamorphic processes. Also called "hydrothermal alteration" it is metamorphism as a result of exposure to hot fluids passing through permeable rocks. Fluids leaving the magma itself causes it, but also ground water seeps down from the surface and is heated by the igneous rocks and then rises because it is hot and carries dissolved minerals upward to form hydrothermal veins. The veins can be rich in precious metals or can grow crystals such as quartz, garnets, and even emeralds. Hydrothermal laboratory methods are used to make synthetic gems under conditions similar to how it happens in the earth. The phrase chemically active fluids is perhaps better than the term hydrothermal because fluids that can changed materials into metamorphic rocks might neither have been water, nor necessary hot (as in boiling). Other "fluid" agents associated with "hydrothermal metamorphism" include carbon dioxide, sulfur oxides, methane and other hydrocarbons. These waters are best described as "brines" because of the high concentrations of dissolve materials they contain. |
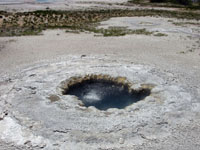
Fig. 11-24. Hot water and gases are highly chemically reactive and will alter rocks they come in contact with. |
11.11
Dynamic Metamorphism
Dynamic metamorphism is metamorphism resulting exclusively or largely from rock deformation, principally folding and faulting. Dynamic metamorphism can take place slowly, such as the gradual bending of a fold in a rock (example in Figure 11-25). Dynamic metamorphism can take place in association with other forms of metamorphism described above, or it can also happen catastrophically, such as within an earthquake fault zone (where shearing of rock take place), or in association with an explosive volcanic eruption or an asteroid impact (Figure 11-26).
The geologic history of our planet is punctuated by many great catastrophic events, none of which have occurred (fortunately) in historic times. Earth has been hit by large asteroids, producing massive craters and causing mass extinctions such as one about 65.5 million years ago that is associated with the disappearance of dinosaurs, ammonites, and many other organisms both on land and within the oceans. Many ancient asteroid impact sites are host to unusual minerals. For instance, microscopic diamonds and other rare minerals have been found in association with ancient asteroid impact sites. Rocks around impact craters and within or around fault zones are sometimes highly shattered or crushed into new metamorphic forms of rocks.
In addition to asteroid impacts, large earthquakes and explosive volcanic eruptions can shatter and alter rocks and result in metamorphism taking place during and following events as fluids have new passageways to migrate through the zone of disruption. |
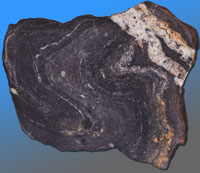
Fig. 11-25. This slab of gray schist shows dynamic metamorphism displayed by evidence of folding with fractures filled with quartz and feldspar (sample also can be called migmatite). |
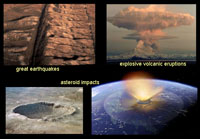
Fig. 11-26. Asteroid impacts, earthquakes, and volcanic eruptions can shatter rocks and cause metamorphism in a variety of ways. |
11.12
Retrograde Metamorphism
Retrograde metamorphism is classic metamorphism happening somewhat in reverse. Retrograde metamorphism involves mineralogical changes that take place when rocks formed at great depths migrate to the surface via tectonic uplift and are exposed to lower pressure and more fluid-rich geologic settings. This causes some minerals to change to match surficial geologic environments. For example, serpentinite forms from the alteration rocks of ultramafic composition (protoliths of mafic igneous rocks pyroxenite and peridotite). These dense rocks are stable in mantle depths, but as they are carried to the surface they expand, possibly remelt, cool, and become exposed to modified seawater seeping into the upper ocean crust. The original minerals (olivine and pyroxene, etc.) expand and their crystal structures change into new serpentine minerals (of which there are many). This expansion lowers their density and they become softer and can flows like plastic under pressure, being ejected upward through zones of weakness in the crust. Pieces of serpentinite often display fluid-flow-like textures (Figure 11-27—this sample is from California's Santa Cruz Mountains). In parts of the Coast Ranges of California there are places where serpentinite flows under pressure and penetrates upward to the surface and flows, much like a slow volcanic eruption, except the rocks are cold. |
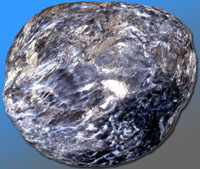
Fig. 11-27. Serpentinite facoid - a naturally polished watermelon -seed-shaped rock displaying plastic-like flow-texture pattern on it surface. |
11.13
Effects of Metamorphism Are Cumulative
In some regions, rocks have been subjected to multiple stages of metamorphism. Through geologic time bodies of rocks within the Earth can be subjected to changing stress fields, moving, rising, sinking, breaking, being heated, cooled, an so on. The older the rock, the more likely it has been exposed to different metamorphic processes. All solid materials have a history, some of which can be deciphered (Figure 11-28). In many cases it is practically impossible to decipher all the changes that have taken place or from what or where the rock originated. |
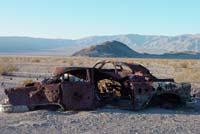
Fig. 11-28. Every solid object has a history. |
11.14
What is the relationship between metamorphism and plate tectonics?
Metamorphism occurs regionally along the subduction zone and also near an igneous intrusion (contact metamorphism). The arrows in Figure 11-29 illustrate compressional forces are coming at each other represent the differential stress and can lead to foliation of metamorphosed rocks.
The movement of tectonic plates transports sediment and rocks into different geologic setting—these changes can result in metamorphism, particularly in zones where tectonic plates are converging, as in a subduction zone or where continental plates converge, pushing up high mountain ranges while material below the mountains are pushed down under increasing temperature and pressure condition (Figure 11-29). These are large scale regions that experience a wide range of conditions through time, and is called regional metamorphism. Areas that are subjected to definable conditions, in part based on the minerals formed, are called metamorphic facies (Figure 11-30). Examples include:
moderate pressure + low temperature = greenschist facies(see Figure 11-32)
high pressure + low temperature = blueschist facies (see Figure 11-33)
moderate pressure + high temperature = amphibolite facies (see Figure 11-34)
high temperature + highest pressure = eclogite facies (see Figure 11-35)
highest temperature + high pressure = granulite facies (see Figure 11-36)
Even though the rock material may have the same chemical composition, their mineral compositions, texture, and appearance are different, and the rocks they comprise are classed into rocks of different metamorphic grades (metamorphic facies). Over the past century, scientists have studies the distribution and occurrences of minerals in the field as well as having manufactured them in laboratories settings, simulating the pressures and temperatures within the earth where different grades of metamorphism takes place. As a result, the temperature and pressure ranges of formation (and destruction) of many minerals, and the metamorphic rocks they form, are well known (Figure 11-31).
Examples of rocks from different metamorphic facies are illustrated below (Figures 11-32 to 11-36). It is important to note that the rocks may have been exposed to the different grades of metamorphism, but the minerals present in those rocks are based on the elemental composition of the protolith from which it formed. For example, a metamorphic rock formed in the amphibolite facies (or amphibolite grade) may or may not contain the mineral amphibole (a common mafic mineral). It may not contain amphibole if there wasn't enough mafic mineral component in the original protolith from which it formed, but it has experienced heat and pressure in the range where amphibolite forms.
Eclogite is a high-grade metamorphic rock containing granular minerals, typically red garnet mixed with grains of pyroxene, quartz, and feldspars and other metamorphic minerals formed under great pressure (Figure 11-35).
Granulite is a very high-grade metamorphic rock containing granular minerals, typically red garnet mixed with grains of pyroxene, quartz, and feldspars formed under great heat without melting (Figure 11-36). |
Fig. 11-29. Generalized illustration of a convergent plate boundary showing regional metamorphism.
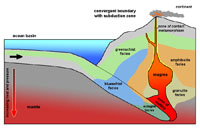
Fig. 11-30. Metamorphic facies in Plate Tectonics
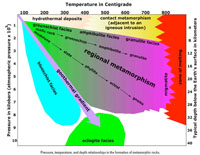
Fig. 11-31. Temperature and pressure effects on metamorphism |
11.15
Examples of rocks from the 5 major metamorphic facies |
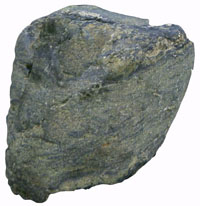 |
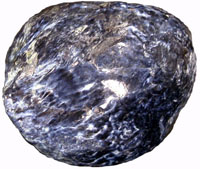 |
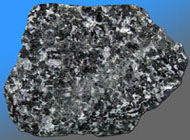 |
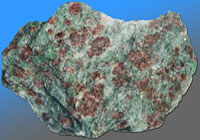 |
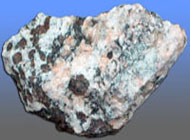 |
Fig. 11-32. Greenschist |
Fig. 11-33. Blueschist |
Fig. 11-34. Amphibolite |
Fig. 11-35. Eclogite |
Fig. 11-36. Granulite |
11.16
Rock Textures Associated With Metamorphism
Metamorphic rocks are typically the hardest to clearly identify for a variety of reasons. They are not only identified by their mineral composition but perhaps as important by the appearance of their texture. Their composition and their texture are related to their origin through metamorphic processes. Regional metamorphism occurs in stages as rocks undergo ever-increasing pressure and temperature changes with burial, and the reverse during uplift and exposure to erosion.
Foliation
Directed pressure reorients minerals with linear or platy structure or to create a preferred orientation of the new mineral grains as they form. Thus elongate minerals such as amphiboles, or platy minerals such as clays or micas tend to align themselves parallel to each other when under pressure. This only happens when there is directed pressure; confining pressure does not accomplish it. A texture of this sort in a metamorphic rock is called foliation (Figures 11-37 to 11-39).
Foliation is any penetrative planar mineral-alignment fabric present in rocks, especially rocks affected by regional metamorphic compression typical of orogenic belts (regions where mountains form from compressional forces in the crust). In foliated rocks, the sheets of mica (or other platy or fibrous minerals) are all oriented in the same direction. Think about how pressure on a house of cards could collapse it under pressure and the card’s flatness would align them in a parallel plane. This texture of many parallel minerals makes a schist and we call the texture foliate texture or a foliated rock. So schist has a foliated texture similar to wood grain, or think also of other things like a deck of playing cards with the edges all lined up, etc.
Crenulation is a cleavage fabric formed in metamorphic rocks that have experience two or more changes in stress directions, resulting in superimposed foliations (Figure 11-40). Rocks including phyllite, schist, and gneiss often display crenulation that has the appearance of small, tight folds, or a wrinkled appearance of layers (note that it is foliation, not bedding!). Foliated texture is extremely important to recognizing regionally metamorphosed rocks and you must be sure to carefully examine the rocks that display it.
Rock cleavage is the capacity of a rock to split along certain parallel surfaces more easily than along others, such as bedding planes. Not all rocks have rock cleavage, but it is perhaps most common in low-grade to moderate-grade metamorphic rocks with a high mica content, such as slate or phyllite where crystalline structure of platy or sheet silicate minerals have been reoriented by metamorphic processes in an alignment perpendicular to the principle vector direction of stress caused by compression (Figure 11-41).
Slaty cleavage is a variety of rock cleavage formed by the reorientation and alignment of clay and mica minerals in fine-grained sedimentary rocks by compaction (high confining pressure) that results in a narrow splitting properties of slate (Figure 11-42). The splitting (slaty cleavage) forms perpendicular to the principle vector direction of stress in the metamorphic setting.
Schistosity the type of rock foliation that characterizes schist, resulting from the parallel arrangement of coarse-grained platy minerals, such as micas (biotite and muscovite), chlorite, and talc (Figure 11-43).
Gneissic texture is a foliated texture in which the coarsely grained mineral crystals have been segregated into discontinuous bands—each band is dominated by one or two minerals, giving the rock a striped appearance . When a granite is subjected to directed pressure, its minerals re-align themselves to adjust to the pressure, forming granite gneiss (Figure 11-44). Schist will also alter into gneiss with increasing heat pressure. this causes segregation into light and dark mineral bands. Gneiss may form by gradual change of schist, but also forms by increased metamorphism of other rocks. It may be difficult to tell from a hand specimen whether a gneiss sample formed from the recrystallization of a granitic protolith, or from the series of changes when sedimentary rocks experience high-grade metamorphism (as illustrated in Figure 11-5). Shale changes to schist and then can be further changed to gneiss.
Distinct Metamorphic Rock Textures
Migmatite is rock intermediate between metamorphic and igneous in character. Migmatite shows irregular banding and much recrystallization, typical of metamorphic gneisses and schists that have taken on igneous character through partial melting. Migmatite commonly show numerous small, light-colored bands of igneous rock cutting through darker foliated schists or gneiss (Figure 11-45).
Mylonite is a fine-grained metamorphic rock, typically banded, resulting from the grinding, shearing, or crushing of other rocks in a fault zone (Figure 11-46). Fault zones are places where fluids can move through, enhancing the processes of metamorphism.
Breccia is a rock consisting of angular rock fragments cemented together (Figures 11-47 and 11-48).
Porphyroblast is a large crystal surrounded by a finer-grained matrix in a metamorphic rock. Porphyroblast are three-dimensional crystals form by the recrystallization at the expense of other minerals during metamorphism. Garnet is perhaps the most common mineral that forms porphyroblast, often having perfect euhedral crystal symmetry that breaks up the parallelism of other platy or parallel growing minerals (Figure 11-49). As porphyroblast form the foliation is not necessarily getting better as larger new minerals grow and the rock changes.
An augen is a large mineral grain (porphyroblast) or grain cluster having the shape of an eye in cross section found in foliated rocks (schists or gneisses). The augens form when large mineral crystals are sheared and deformed during metamorphism (Figure 11-50).
|
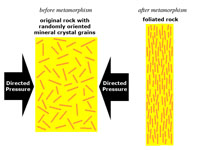
Fig. 11-37. Foliation forms from the recrystallization (realignment) of minerals into bands that are perpendicular to the force of directed pressure. |
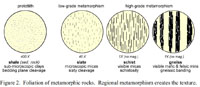
Fig. 11-38. Foliation in metamorphic rocks develops in stages. Directed pressure associated with regional metamorphism creates these textures. |
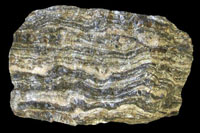
Fig. 11-39. Foliation is illustrated in this piece of gneiss. Light bands are quartz and feldspars, dark bands are biotite and mafic minerals. |
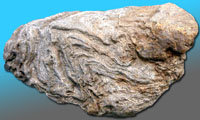
Fig. 11-40. Crenulation is tight small scale folding of mineral bands, common in metamorphic rocks that have experienced more than one stage of metamorphism. |
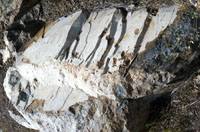
Fig. 11-41. Rock cleavage illustrated in this outcrop of metavolcanic rock. |
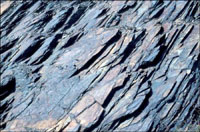
Fig. 11-42. Slaty cleavage (a form of rock cleavage as illustrated by slate). |
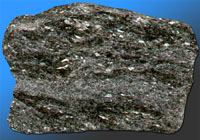
Figure 11-43. Schistosity (as illustrated of this specimen of biotite schist) |
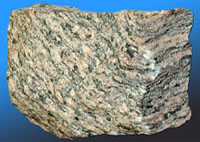
Fig. 11-44. Gneissic texture (as illustrated in this piece of granite gneiss). |
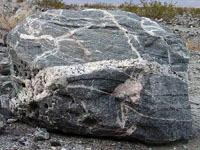
Fig. 11-45. Migmatite (as illustrated by this boulder of dark gneiss with light-colored veins of pegmatite and aplite dikes). |
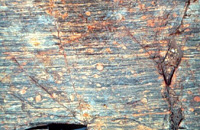
Fig. 11-46. Mylonite (stretched and fractured blocks of gneiss and schist) exposed in the Grand Canyon inner gorge. |
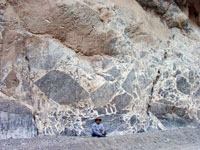
Fig. 11-47. Breccia in marble exposed in Death Valley National Park, CA |
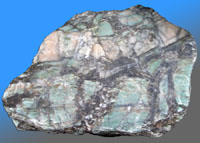
Fig. 11-48. Green, white, and gray chert breccia (metachert) |
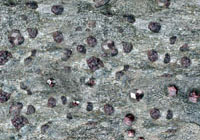
Fig. 11-49. Garnet porphyroblasts in a matrix of mica schist. |
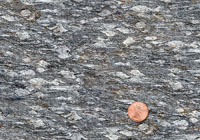
Fig. 11-50. Augens (sheared feldspar porphyroblasts) in gneiss. |
11.17
Non-Foliated Metamorphic Rocks
Some metamorphic rocks do not display foliation. Rocks that have been subjected to high heat but relatively low to moderate amount of directed pressure may not display foliation (realignment of crystals). Examples of metamorphic rocks that commonly lack foliation include marble, quartzite, and amphibolite.
Marble is metamorphosed limestone. Limestone is an accumulation of calcite (CaCO3; bubbles with acid), it may be from calcareous skeletal remains (shells, algal remains, etc.) or from inorganically precipitated evaporite-type deposition of sedimentary origin. Whatever its original source, limestone when heated or put under pressure may recrystallize (the calcite crystals enlarge to visible grains) and form a coarse crystalline rock called marble. Marble may be white, pink, blue, or even dark colors. What distinguishes marble from limestone is the coarseness of the calcite crystals and the fact that any fossil remains are destroyed by the process of recrystallization (Figure 11-51).
As the limestone recrystallizes, impurities may provide a source for the formation of garnets, tourmaline, spinel, and most importantly ruby; other gem minerals may occur as well. Though marble is a good example of a rock that can form from contact metamorphism, not all marble forms this way. In mountainous regions, limestones may undergo directed pressure where regional metamorphism occurs. Thus to interpret a marble’s origin, you must see if the other rocks near it are foliated or not.
Marbles are usually made of calcite or dolomite which has two important properties that effect the rocks appearance. The minerals calcite and dolomite forms rhombohedral crystals which easily recrystallize under heat and pressure. It is not easy to foliate marble (Figure 11-52). To determine if the marble is composed of calcite or dolomite, calcite will bubble if acid is applied, but sometimes they are made of a related mineral called dolomite which only bubbles if it is first ground to a powder and then has acid applied.
|
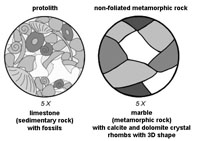
Fig. 11-51. Marble is created by changing limestone (protolith) by recrystallization. The fossils are destroyed. Contact or regional metamorphism can do this.
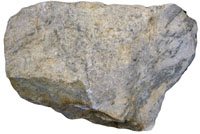
Fig. 11-52. Dolomite marble from the Gavilan Range in California. |
11.18
Metamorphism of Organic Materials
This section briefly describes the role of metamorphism of organic materials in the formation of oil, gas, and coal.
Trophic respiration involves all processes in organisms that result in release of energy related to the consumption of substances that go through a chemical changes. These processes may result in the excretion of substances that can alter and/or accumulate in the environment (Figure 11-53). This includes organisms after they die (if their remains are not completely consumed by other organisms). Organic remains are highly susceptible to chemical changes under increasing temperature and pressure with burial.
Organic Maturation
Many processes take place in the processes that change dead organic matter into energy resources like coal, oil and gas. These changes take place as sediments containing organic material undergoes increasing burial—involving increasing heat and pressure through geologic time. The changes that take place are called organic maturation and are similar to the processes that take place when food is cooked in an oven. Organic maturation is the gradual metamorphic processes that take place over time, involving burial and geothermal heating, that convert organic remains preserved in sediments into petroleum (oil, gas, and tar) or coal (conversion of plant material to peat, lignite, sub-bituminous coal, bituminous coal, and anthracite coal, in increasing order of maturation)(Figures 11-54 to 11-56). Rocks containing organic matter go through stages of organic maturation, even to the point of being over toasted or burned—causing organic materials to break down, driving off their volatile components (including methane, CO2, ammonia, water and other gases) leaving behind nearly pure carbon residues. Tars and asphalt are highly enriched in carbon (Figure 11-55). |
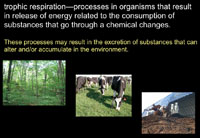
Fig. 11-53. Trophic respiration produces organic residues (plant & animal tissue, bone, fecal waste, etc).
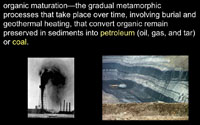
Fig. 11-54. Oil and gas, and coal all form by organic maturation |
11.19
Origin of Coal
In general, coal forms from the remains of land plant materials that are rich in carbohydrates, particularly organic materials called lignins and cellulose derivatives. Coal forms from accumulation of plant materials is swampy environments with stagnant water conditions (oxygen is depleted so organic compound can survive aerobic decay). With time, burial, and compaction, plant material becomes peat, then lignite, then coal (first bituminous coal then anthracite coal)(Figure 11-56). Anthracite can be argued to be a low-grade metamorphic rock. (The gems called jet is a very pure carbon variety of anthracite coal.) The effect increasing heat and pressure results in organic (volatile) compounds being driven off while the residue becomes enriched in carbon. The volatile compounds include natural gas (mostly methane, CH4), CO2, water, other natural gases and acids (basically compounds containing hydrogen, oxygen, nitrogen, and others are driven off while the carbon remains behind, becoming coal. Note that in Figure 11-56 that the organic materials become increasingly black as metamorphism proceeds.
Stages in the conversion of plant material into coal (Figure 11-56). |
Peat—an accumulation of partially decayed vegetation matter that has a brown, soil-like character typical of boggy, acid ground or swampy setting.
Lignite—an organic deposit of soft brownish coal preserving traces of plant structure, intermediate between peat and bituminous coal.
Bituminous coal—soft black coal with a high volatile content, and typically burns with a smoky yellow flame.
Anthracite—a hard, metamorphic variety of coal, having a low volatile content. Typically burns very hot and clean relative to other varieties of coal. |
|
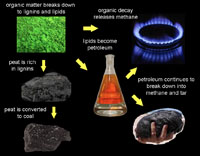
Fig. 11-55. petroleum and coal from from organic residues.
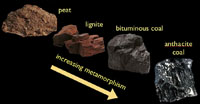
Fig. 11-56. Formation of coal from plant material through organic maturation. |
11.20
Origin of Petroleum (Oil & Gas)
Petroleum is a natural flammable liquid mixture of hydrocarbons that is present in certain rock strata and can be extracted and refined to produce fuels including gasoline, kerosene, diesel oil, or chemically converted to other materials, such as plastics, and other petroleum-based byproducts. Oil is the liquid component of petroleum (as opposed to gas or asphalt or tar). Petroleum is the derivative of the metamorphism of organic-rich sedimentary rocks rich in volatile components, especially lipids. Natural gas includes hydrocarbons that are in gaseous state under normal atmospheric pressures.
Petroleum undergoes the same degrees of metamorphism with increasing heat and pressure over time. Most oil is derived from source rocks that are rich in bitumens—mostly oils and waxes (hydrogen-rich organic compounds). Marine plankton, particularly single-celled plants, are have higher concentrations of these compounds that eventually end up buried in sediments on the sea bed. Although animal remains are rich in rich in these compounds, volumetrically their remains are a minuscule fraction of the organic residues preserved in sediments. As petroleum residues mature they undergo the same process of separation of the hydrogen-rich and carbon-rich compounds. Heat causes oils to break down to the ultimate end-members of hydrogen-rich natural gas (mostly methane, CH4) and carbon-rich molecules associated with tars and asphalt (which can be hard as a rock).
Characteristics of a Petroleum Reservoir (oil &gas) |
Figure 11-57 shows what the geometry of a petroleum reservoir can look like in the subsurface. A reservoir is a subsurface pool of hydrocarbons contained in porous or fractured rock formations. Oil and gas float on water and fills in pore space in sediments as water is displaced. Gas is less dense and floats on top of oil. A reservoir needs a "cap rock" layer that acts as a barrier to prevent oil and gas from migrating to the surface. The reservoirs illustrated in Figure 11-57 are anticlines with the reservoir rock being porous sandstone, and the caprock are a layers of impermeable shale. A reservoir also need source rocks. Source rocks are sedimentary rocks rich in organic residues that with enough heat and time releases their volatile components, allowing them to migrate to other locations, including the surface.
Fracking is a new technology used to extract petroleum directly from source rocks that have not released their petroleum because the oil and gas components are trapped in confined pore spaces in fine-grained sediments. A well is drilled into a layer of source rock, and explosive charges are set off to fracture the rock. Chemicals are pumped into the rock to force open the fractures and to help release to petroleum directly from the source rocks (Figure 11-58 illustrates a traditional oil & gas well with the newer method of fracking extraction well.) |
Crude oil that is sent to a refinery undergoes a heating and distillation process similar to what takes place naturally underground as rocks bearing organic matter undergo maturation over time. In a petroleum refinery, as the crude oil is heated, different organic derivatives boil, separate, and condense at different temperatures (Figure 11-59). Volatile hydrocarbon compounds rich in hydrogen separate from those rich in carbon. Underground, over time these different components separate into gas, oil, and tar.
|
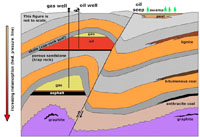
Fig. 11-57. Organic maturation of petroleum and coal through increasing metamorphism. Coal is illustrated on the right. The left side illustrates petroleum reservoirs. With increased metamorphism, oil will break down to form natural gas (rich in hydrogen) and tar residues (rich in carbon).
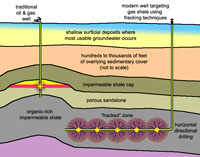
Fig. 11-58. Traditional oil well and a shale fracking well.
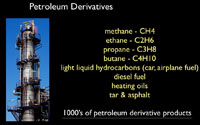
Fig. 11-59. Petroleum products are distilled from crude oil in oil refineries. |
11.21
Graphite
Graphite is a high-grade metamorphic mineral composed of pure carbon, possibly formed from the metamorphic decay of hydrocarbons. However, carbon in graphite deposit may or may not be directly of organic origin, it may come from other sources such as carbon-bearing gases released from magma or from the extreme metamorphism of carbonate minerals (such as calcite or dolomite) which easily lose their CO2 when heated. However, even the carbon in magma and certainly limestone and marble is probably of organic origin deep in the past. Graphite is abundant in some high-grade metamorphic rocks (Figure 11-60). In contrast, the carbon that forms diamonds is from deep in the mantle (where much greater pressure and temperature conditions exist), but whether that carbon in diamonds was once organic residue from the earth surface in the distant past is unknown.
Are Diamonds Of Organic Origin?
A discussion of the metamorphism of organic remains has some bearing on the formation of diamonds. Most diamonds are carbon mineral derived from igneous sources deep in the upper mantle (discussed in Section 7.24 in Chapter 7). They have also been found in association with deposits of large asteroid impacts. Despite mythology, diamonds do not form from coal (except when squeezed in the hands of Superman, of course!). |
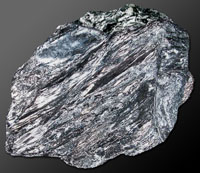
Fig. 11-60. Graphite is a soft mineral of pure carbon, just like diamond, but with a different crystalline structure. |
|